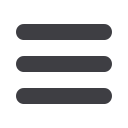

30
Chapter 2
no effect in the group of subjects with already high dopamine levels. Furthermore, a number
of studies have shown that the effects of a dopamine D2 receptor agonist can be explained by
natural variation in the gene coding for the dopamine D2 receptor (Kirsch et al., 2006; Cohen
et al., 2007). Subjects carrying the
Taq
1A1 variant of the allele (A1+ subjects) have ~30%
fewer dopamine D2 receptors in the striatum and exhibit impairments in reward processing,
compared with those not carrying this allele (A1- subjects). Cohen and colleagues (2007)
assessed whether this genetic predisposition could explain individual responses to a dopamine
receptor agonist during reward processing. To this end they used a reversal learning paradigm,
which requires flexible adaptation of behaviour when a previously rewarded stimulus is no
longer rewarded and a newrule needs tobe learned. In the placebo condition, the lowdopamine
receptor group (A1+ subjects) performed worse than the A1- group. However, administration
of the dopamine D2 receptor agonist cabergoline improved rule-learning performance in the
subset of subjects with genetically determined low dopamine receptor density (A1+), but
the dopamine D2 receptor agonist impaired performance in those already performing well
under placebo (the A1- group). This effect was accompanied by opposite effects in reward-
related neural responses in regions of the reward network (the medial orbitofrontal cortex
and striatum): Administration of the D2 receptor agonist increased activity in these regions in
subjects with low reward-related activity under placebo (A1+), while it had the opposite effect
in those with already high baseline reward-related activity (A1-).
As was discussed in
chapter 1
, in addition to predicting individual differences indrug response,
natural (genetic) variation between individuals can also explain individual differences in task
performance (e.g. (Frank et al., 2007; Dreher et al., 2009; Aarts et al., 2010; Colzato et al.,
2010a; Stelzel et al., 2010) (
box 2.2c
). In
chapters 3 and 4
, I exploited individual variability
in the gene coding for the dopamine transporter (DAT) to account for inter-individual
variability in task performance, neural signalling, and drug response. Task-related differences
in performance or neural signalling as a function of variation in this genotype can be taken to
suggest that dopamine is involved in the studied process.
Evidence for a role for dopamine in the integration of reward motivation and cognitive control
has been provided by a number of studies, and much of this evidence is reviewed in
chapter
1
of this thesis. Previous work that also employed the rewarded task-switching paradigm
presented in this thesis (
box 2.3
) has shown that reward can modulate flexible control in
the context of task switching (Aarts et al., 2010). The anticipation of a reward (i.e. high vs.
low reward cue) increased neural responses in the ventral striatum, while the integration
between reward and task switching was associated with increased signalling in the caudate
nucleus. Interestingly, these signals correlated, suggesting that communication between the
ventral and dorsal striatum may mediate the information transfer from reward regions to
cognitive control regions (
figures 1.2c, d and 2.1, box 2.1
). Dopamine-dependent effects in
this latter study were revealed by showing that inter-individual differences in signalling in
the caudate nucleus depended crucially on individual differences in dopamine signalling,
measured by exploiting differences in the
DAT1
genotype. Although these results provide