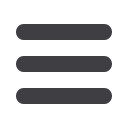

109
5
Especially when fitting recipients, which cannot give clear responses, it is valuable to know that Tand
M-levels tend to increase over time, as shown in figure 3. This finding was partly confirmed by previous
research [Hughes et al., 2001; Henkin et al., 2006; Walravens et al., 2006; Smoorenburg, 2007; Zwolan et
al., 2008; Wesarg et al., 2010]. These rising levels over time could be explained by new intracochlear fibrous
tissue formation [Somdas et al., 2007] or by increased behavioral loudness tolerance [Hughes et al., 2001].
Interestingly, no significant increase in electric DR was shown over time if it was expressed in decibels,
whereas it evidently increased if it was expressed in clinical units. This relates directly to the ongoing debate
whether electrical stimulation levels should be expressed in linear units or on a logarithmic scale. The
loudness theory of electrical stimulation proposed by Zeng and Shannon [1994] would be better suited
with a linear current scale. Kwon and van den Honert [2006], however, argue that a logarithmic scale
would match better with subjective loudness growth when dealing with larger electric stimulation ranges.
Moreover, the fact that, in clinical practice, levels are expressed in both logarithmic (Cochlear) and linear
scales (MED-EL, Advanced Bionics), makes it difficult to compare published data between manufacturers.
In our data, an average increase of 1.7–1.8 dB over 1 year was evident for both Tand Mlevels, whereas
increases, if expressed in clinical units, differed considerably between T-levels (11.0 CU) and Mlevels (40.6
CU). Apparently, expressing fitting levels in decibels facilitates the translation of our findings to clinical
practice.
The data plotted in figure 4 show the significant correlation between speech perception and T-levels (r =
0.34, p < 0.01) and DR (r = 0.33, p < 0.01), respectively. This underlines the value of setting appropriate
T-levels in an individual patient. However, our findings support the use of a preset profile for the M-levels.
Firstly, M-levels were not significantly correlated with speech perception. Secondly, the use of the preset
M-level profile with emphasis on the higher frequencies led to speech perception scores (on average 57%
words correct in a monosyllabic word test) which are in line with word scores in the recent literature (65%
in Holden et al. [2011]; 50% in Finley et al. [2008]). This is contrary to the use of a flat M-level profile,
as this was shown to negatively influence speech understanding [Boyd, 2010]. Nevertheless, other factors
are expected to be larger contributors to speech perception. Although not confirmed by all research groups
[Roditi et al., 2009], duration of deafness was repeatedly shown to be a predictor of speech perception,
with up to 30% of the variance explained by this single factor [Gantz et al., 1993; Waltzman et al., 1995;
Rubinstein et al., 1999; Friedland et al., 2003; Gomaa et al., 2003]. In the present cohort, this effect is
much less prominent – although still significant – with, on average, a 3% reduction in monosyllabic word
score per decade of deafness. A similar effect was found for age at implantation.
Figure 5 shows that some other factor (or factors), but not the overall level, causes an increase in levels
towards the basal end of the electrode array, and this increase turned out to be level independent when
levels were recalculated from clinical units to decibels. An increase in T-levels towards the basal end was
reported by more researchers. Some authors blame an offset towards the base on the increased distance
to the modiolus [Gordin et al., 2010], others ascribe it to new bone formation [Fayad et al., 2009] or a