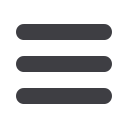

FROZEN HEAT
36
here on continental margin settings, which combine the
appropriate pressure and temperature conditions for meth-
ane hydrate formation with regions of high sedimentation
rates and elevated “primary productivity,” which is the
rate at which organic carbon is produced in surface waters
(Reeburgh 2007). As discussed in Volume 1, Chapter 1, the
rapid burial of organic carbon that “rains” to the sea floor
can promote microbial breakdown of that organic mate-
rial, with methane as a key by-product. At the appropriate
pressure and temperature conditions, this methane can be
incorporated into methane hydrates within the sediments,
usually at water depths greater than ~500 m. Because the
combination of high primary productivity and high organic-
carbon burial rate is mostly confined to continental margins
(Hedges and Keil 1995; Buffett and Archer 2004), continen-
tal margins host most of the world’s gas hydrate while sedi-
ments of deep ocean basins are relatively free of methane
hydrate, even though the deep-ocean pressure and tempera-
ture conditions are suitable for gas-hydrate formation (See
Volume 1 Chapter 1).
Methane dissolved in pore water and sulphate are biologically
converted to bicarbonate, hydrogen sulphide and water
Methane and oxygen are biologically and chemically
converted to carbon dioxide near the sea oor
Methane and oxygen are biologically and
chemically converted to carbon dioxide
Anaerobic oxidation of methane
Aerobic methane oxidation
Aerobic methane oxidation
Methane chemically reacts to form many
compounds, including carbon dioxide
Aerobic methane oxidation
Zone 1
Zone 2
Zone 3
Zone 4
Methane consumption in the environment
Oxygenated sediments
Water column
Atmosphere
Anoxic sediments
Gaseous methane can bypass the sediment-
based bio lter by migrating along permeable
paths, such as faults
Where methane rises from
the sea oor in plumes of
bubbles, much of the
methane dissolves before
reaching the surface
Methane di solved in pore water and sulphate are biologically
converted to bicarbonate, hydrogen sulphide and water
Methane and oxygen are biologically and chemically
converted to carbon dioxide near the sea
or
Methane and oxygen are biologically and
chemically converted to carbon dioxide
Anaerobic oxidation of methane
Aerobic methane oxidation
Aerobic methane oxidation
Methane chemically reacts to form many
compounds, including carbon dioxide
Aerobic methane oxidation
Zone 1
Zone 2
Zone 3
Zone 4
et a e c s ti i t e e vir
e t
Oxygenated sediments
Water column
Atmosphere
Anoxic sediments
Gaseous methane can bypa s the sediment-
based bio lter by migrating along permeable
paths, such as faults
Where methane rises from
the sea
or in plumes of
bu bles, much of the
methane di solves before
reaching the surface
Figure 2.3:
Methane consumption in the environment. Near sea-floor methane hydrate is being continuously broken down, releasing
methane dissolved in pore water. As methane moves through sediment into the water column and atmosphere, it is consumed in a variety
of chemically and microbially controlled reactions. As listed on the left, dissolved-phase methane can then be consumed by microbes
as part of an extended chemosynthetic food chain (see also Fig. 2.7) or consumed chemically. As shown on the right, gaseous methane
can bypass the microbially controlled reactions in the sediment because microorganisms can access only dissolved methane (Treude
et
al.
2005b; Treude and Ziebis 2010). Methane in bubbles entering the water column tends to dissolve into the water, where it can then
be consumed by aerobic microbes. The methane “biofilter” removes much of the methane that would otherwise be transported into the
atmosphere. Figure is not drawn to scale. For hydrates in the marine environment, the water depth (Zone 3) would generally be 300-500
metres or more, Zone 2 would be on the order of 1 centimetre thick, and Zone 1 would be on the order of 10 metres thick.