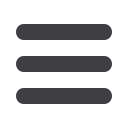

Engineering Approaches to Biomolecular Motors: From in vitro to in vivo Thursday Speaker Abstracts
20
Engineering Inhibitable Kinesin Motors
Kristen Verhey
.
University of Michigan, Ann Arbor, MI, USA.
The human genome encodes 45 kinesin motor proteins that drive cell division, cell motility,
intracellular trafficking, and ciliary function. Determining the cellular function of each kinesin
would benefit from specific small molecule inhibitors. However, screens have yielded only a few
specific inhibitors. Here we present a novel chemical-genetic approach to engineer kinesin
motors that can carry out the function of the wildtype motor yet can also be efficiently inhibited
by small, cell-permeable molecules. Using kinesin-1 as a prototype, we developed two
independent strategies to generate inhibitable motors, and characterized the resulting inhibition
in single molecule assays and in cells. We further applied these two strategies to create
analogously inhibitable kinesin-3 motors. These inhibitable motors will be of great utility to
study the functions of specific kinesins in a dynamic manner in cells and animals. Furthermore,
these strategies can be used to generate inhibitable versions of any motor protein of interest.
Creating Novel Biomolecular Motors Based on Dynein and Actin-binding Proteins
Akane Furuta
1
, Kazuhiro Oiwa
1,2
, Hiroaki Kojima
1
,
Ken'ya Furuta
1
.
1
NICT, Kobe, Japan,
2
University of Hyogo, Harima Science Park City, Hyogo, Japan.
Biomolecular motors have the potential to be used as molecular-scale actuators, switches, and
robots in nanoscale devices. Protein engineering is a key technology for such applications;
however, it is currently not possible to design a novel biomolecular motor from scratch. Here, we
present an alternative strategy where the existing functional modules (as protein building blocks)
are combined through protein engineering techniques such as domain swapping and circular
permutation to create a new series of biomolecular motors. According to the new bottom-up
strategy, we successfully created novel actin-based motors; we show that the hybrid motors —
combinations of a motor core derived from the microtubule-based dynein motor and non-motor
actin-binding proteins — robustly drive the sliding movement of an actin filament. Furthermore,
the direction of actin movement is reversible by simply changing the geometric arrangement of
these building blocks. Our results emphasize that our strategy is useful for rapidly obtaining
biomolecular motors with desired properties. At the same time, the new strategy combined with
structural studies can be a powerful tool to investigate the design principles of biomolecular
machines.