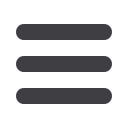

ESTRO 35 2016 S759
________________________________________________________________________________
1
The Netherlands Cancer Institute, Department of Radiation
Oncology, Amsterdam, The Netherlands
Purpose or Objective:
Labour-intensive procedures, such as
adaptive radiotherapy and the upcoming new modalities
protons and MR linac, result in an increased workload in the
treatment planning department. We therefore started the
FAST-planning project, a Framework for Automatic
Segmentation and Treatment planning. The purpose of this
project is to produce single-click automated treatment
planning for the majority of tumour sites.
Material and Methods:
Easy configuration of treatment
protocols was achieved by isolating medical planning protocol
relations from software: in-house developed XPP document
format (eXtensible Planning Protocol) allows for a complete
planning protocol definition in a single document (XML). In
FAST planning, the patient ID, dicom identifiers and the
selected planning protocol are combined, and an Autoplan
document (XML) is composed.
In the framework, each module accepts Autoplan documents
and coordinates actions accordingly; e.g. automatic
localization of the patient record, import of DICOM objects
with delineated target volumes, auto-segmentation of OARs,
creation of additional ROIs, creation of advanced beam-
setups (VMAT, IMRT), optimization and finally the creation of
a report (optionally uploaded to R&V MOSAIQ). The software
is written in Python and makes use of Pinnacle3 scripting and
transfer protocols DICOM and XML over HTTP. Schemas are
used for validation of all XML documents.
Results:
The following workflow is automated: after the
physician delineated the target, a single mouse-click initiates
RT plan generation on our remote treatment planning system
Pinnacle3. Subsequently a preview report of the generated
plan is send to R&V system MOSAIQ (Fig. 1). The created RT
plan is fully optimized and ready for inspection by the
dosimetrist. FAST-planning has been implemented into our
clinic for Breast, Prostate, and Vertebral metastases.
Nine Prostate protocols (VMAT) are in place for a variety of
dose-levels (51, 64.6 and 77Gy) and target definitions
(boost/no-boost and inclusion of seminal vesicles). For
Breast, 8 IMRT plans (variation in beam-setup and OAR
margins) are created; the dosimetrist and physician can
select the best plan based on target coverage and dosimetric
trade-offs. For vertebral metastases, 2 plans (conformal
beam-setups PA and APPA) are created and screenshots in
PDF are sent to R&V MOSAIQ for plan evaluation and selection
by the physician.
Conclusion:
We have introduced fully automated RT planning
for treatment plans Breast (in 20min), Prostate (in 20min)
and palliative Vertebrae (in 7min). The automation of these
treatment sites has reduced the dosimetrist's planning time
considerably (up to 2 hours per RT plan), while maintaining
the same plan quality. The FAST framework is generic and
allows for easy RT planning protocol configuration for the
EBRT techniques VMAT, IMRT and conformal fields. The
workflow automation currently covers approx. 20% of our
patient throughput, i.e. 1250 RT planning sessions/year.
EP-1629
A novel method for electron beam geometry optimisation
T. Felefly
1
Hôtel Dieu de France - Saint Joseph University, Radiation
Oncology, Beirut, Lebanon
1
, C. El Khoury
1
, F. Azoury
1
, N. Farah
1
, J. Barouky
1
,
R. Sayah
1
, N. Khater
1
, D. Nehme Nasr
1
, E. Nasr
1
Purpose or Objective:
A normal beam incidence optimizes
dose distribution in electron radiotherapy. Historically,
electron beam direction is chosen clinically with aid of
Computed Tomography (CT) data, but commonly without
couch rotation. This work describes a novel method for
optimizing electron beam incident angle by varying both
gantry and couch angles.
Material and Methods:
The treated skin surface could be
represented using triangle mesh modeling, the vertices being
chosen as points on the treated body contour, and their 3D
coordinates obtained from the CT dataset. The optimal beam
direction would be parallel to the vector sum of all normal
vectors to the defined triangles. For each triangle, the
normal vector can be obtained by the cross product of two
vectors formed by the triangle vertices.Gantry and couch
rotation angles of the electron field could then be derived
from the vector sum using simple trigonometric formulation.
A computer code based on these formulas was developed.
The inputs required are the vertices 3D coordinates, the
output being the calculated gantry and couch rotation angles.
Ideally, using a larger number of vertices, and consequently a
larger number of triangles, increases the similarity between
the mesh representation and the real skin surface.For
practical reasons, two software versions were generated: one
using four vertices selected on the treatment planning system
such that they are located on the periphery of the treated
skin, and the other using nine points selected on the
periphery and evenly distributed within the treated skin.
Results were compared for fifteen treatment plans and
evaluated clinically in the treatment room and dosimetrically
using the Eclipse Monte-Carlo electron algorithm.
Results:
The two software versions yielded similar results,
the root-mean-square deviation being 1.28° for couch
rotation angles and 1.9° for gantry angles. When assessed
clinically on patients, the derived beam direction appeared
fairly normal to the treated skin surface for all cases. A
better dose distribution was obtained using the software
particularly for cases with large calculated couch rotation
angles.
Conclusion:
This software tool is an alternative to the
historically used method, is more objective and accurate,
may provide a better dose distribution, and is reasonably
practical using the four vertices based calculation.