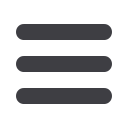

S201
ESTRO 36 2017
_______________________________________________________________________________________________
received an inadequate therapeutic dose. Concerning the
low number of locoregional relapses in AC patients after
definitive CRT one has to balance increased skin side
effects by including the AILD into the standard CTV against
a rigid oncological-anatomical interpretation of the local
lymphatic drainage.
Award Lecture: Company Award Lectures
OC-0376 Trajectory Optimization in Radiotherapy
Using Sectioning (TORUS)
C. Locke
1
, K. Bush
1
1
Stanford Cancer Center, Radiation Oncology, Stanford,
USA
Purpose or Objective
One of the most challenging problems in trajectory
optimization for radiotherapy is properly handling the
synchronization of the medical accelerator’s dynamic
delivery. The initial coarse sampling of control points
implemented in a Progressive Resolution Optimization
type approach (VMAT) routinely results in MLC aperture
forming contention issues as the sampling resolution
increases. IMRT based solutions such as 4Pi avoid MLC
synchronization issues through use of a static gantry, but
inevitably suffer from longer treatment times. This work
presents an appoach to optimize continuous, beam-on
radiation trajectories thorough exploration of the
anatomical topology present in the patient and formation
of a novel dual metric graph optimization problem.
Material and Methods
This work presents a novel perspective on trajectory
optimization in radiotherapy using the concept of
sectioning (TORUS). TORUS avoids degradation of 3D dose
optimization quality by mapping the connectedness of
target regions from the BEV perspective throughout the
space of deliverable coordinates. This connectedness
information is then incorporated into a graph optimization
problem to define ideal trajectories. The unique usage of
two distance functions in this graph optimization permits
the TORUS algorithm to generate efficient dynamic
trajectories for delivery while maximing the angular flux
through all PTV voxels. 3D dose optimization is performed
for trajectories using the Varian’s Photon Optimizer
(version 13.6.23).
Results
The TORUS algorithm is applied to three example
treatments: chest-wall, scalp, and the TG-119 C-shape
phantom. When restricted to only coplanar trajectories
for the chest-wall (dose distributions shown in Figure 1)
and scalp cases, the TORUS trajectories are found to
outperform both 7 field IMRT and 2 arc VMAT plans in
delivery time, organ at risk sparing, conformality, and
homogeneity. When the coplanar restriction is removed
for the TG-119 phantom and the static non-coplanar
trajectories are optimized, TORUS trajectories have
superior sparing of the central core avoidance with shorter
delivery times, with similar conformality and
homogeneity.
Conclusion
The TORUS algorithm is able to automatically generate
trajectories having improved plan quality and delivery
time over standard IMRT and VMAT treatments. TORUS
offers an exciting and promising avenue forward toward
increasing our dynamic capabilities in radiation delivery.
OC-0377 Limited interfractional variabi lity of
respiration-induced tumor motion in esophageal
cancer RT
P. Jin
1
, M.C.C.M. Hulshof
1
, N. Van Wieringen
1
, A. Bel
1
, T.
Alderliesten
1
1
Academic Medical Center, Radiation Oncology,
Amsterdam, The Netherlands
Purpose or Objective
Respiration-induced tumor motion is one of the major
sources of intrafractional uncertainties in esophageal
cancer RT. However, the variability thereof during the
treatment course is unclear. In this study, we investigated
the interfractional variability of respiration-induced
esophageal tumor motion using fiducial markers and 4D-
CBCT.
Material and Methods
We included 24 patients with in total 65 markers
implanted in/around the primary esophageal tumor. Per
patient, a 3D planning CT (pCT) and 7–28 (median: 8) 3D-
CBCTs were acquired. Using the fluoroscopy projection
images of the 3D-CBCTs, 10-breathing-phase 4D-CBCTs
were retrospectively reconstructed. First, for each 4D-
CBCT, the 10 phases were rigidly registered to the pCT
based on the vertebra. Next, each marker in each phase
was registered to its corresponding marker in the pCT to