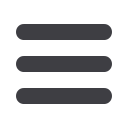

S346
ESTRO 36 2017
_______________________________________________________________________________________________
and 37.5 months in surviving patients, the majority of
patients (90%) have died. One-year overall survival and
local control were 45% and 30% respectively. However,
high-dose SBRT was associated with improved local control
(p < .01), and the one-year overall survival and local
control were 77.8% and 66.7% respectively in this sub-
group. Furthermore, an increased mean interval between
initial treatment and SBRT was observed in patients who
achieved durable local control (41.9 vs. 13.4 months, p <
.01). While treatment was generally well tolerated, there
were two cases of radiation pneumonitis (grade 2) and two
cases of recurrent laryngeal nerve paralysis (grade 2 and
3), all of which resolved prior to last follow-up or death.
No late esophageal toxicity was noted. A patient who
received an SBRT dose of 45 Gy (total EQD2: 129.7 Gy)
experienced cardiopulmonary death 35 months following
treatment which was attributed to radiation toxicity.
Conclusion
Although the overall prognosis for patients with in-field
central NSCLC recurrences following CF-EBRT remains
grim, five-fraction SBRT was well tolerated with an
acceptable toxicity profile. Dose escalation above 35 Gy
may offer improved local control, however caution is
warranted when treating high-risk recurrences with
aggressive regimens. Our findings support the efficacy of
five-fraction SBRT re-irradiation reported by Trovo et al.
[Int J Radiat Oncol Biol Phys. 2014 Apr 1;88(5):1114-9].
PO-0669 Models of pulmonary function changes after
thoracic radiotherapy
A.G.H. Niezink
1
, O. Chouvalova
1
, J.F. Ubbels
1
, A.J. Van
der Wekken
2
, J.A. Langendijk
1
, J. Widder
1
1
UMCG University Medical Center Groningen, Radiation
Oncology, Groningen, The Netherlands
2
UMCG University Medical Center Groningen, Pulmonary
Diseases, Groningen, The Netherlands
Purpose or Objective
Reproducibly measuring pulmonary toxicity remains
challenging in thoracic radiotherapy. Pulmonary function
tests may render objective parameters to assess
pulmonary radiation toxicity. Our aim was to establish a
model predicting post-radiotherapy forced expiratory
volume in one second (FEV1) and diffusion capacity
(DLCO).
Material and Methods
Patients with both baseline and follow-up FEV1 and/or
DLCO available were included from a prospective data
registry (clinicaltrials.gov). Patient and tumour
characteristics as well as dose-volume parameters and
survival data were available. Changes in pulmonary
function tests were calculated using a paired t-test, and
univariable and multivariable linear regression models
were built predicting pulmonary function test changes.
Multicollinearity was tested using the variance inflation
factor and the quality of the models were compared using
adjusted R-square and the Akaike information criterion
(AIC).
Results
Baseline and follow-up FEV1- and DLCO-data were
available for 379 and 283 patients, respectively, who were
treated between 2013 and 2015 for (N)SCLC stage I-III with
(chemo)radiotherapy or SABR. Both FEV1 and DLCO
declined significantly after treatment (p=0.001 and
p<0.001). WHO-performance status (2-3 versus 0-1),
chemotherapy (yes versus no), smoking (never versus
former or current), technique (SABR versus external beam
RT), GTV, lung dose-volume parameters (V5, V20, V30,
V40, mean lung dose) and heart volume parameters (V5,
V40 and mean heart dose) were significant factors
predicting follow-up DLCO after adjustment for baseline
DLCO. The best model, based on multivariable linear
regression for predicting follow-up DLCO, contains
baseline DLCO, WHO-performance status and lung V5
(adjusted R-square=0.71, p<0.0001) [Figure 1].
Univariable and multivariable linear regression showed
that baseline FEV1 and lung V40 are significant factors
predicting follow-up FEV1 (adjusted R square = 0.21,
p<0.0001).
Figure 1
: Baseline DLCO and post-radiotherapy DLCO
decline by V5-lung for three different scenarios.
Abbreviations: DLCO
Fu
and DLCO
BL
= diffusion capacity of
carbon monoxide corrected for hemoglobin concentration
at follow-up and baseline; WHO-PS=WHO performance
score (binary: 2-3 versus 0-1); V5Lung= percentage of lung
volume receiving 5Gy or more. p25 / mean / p75 = 25
th
percentile, mean and 75
th
percentile of the V5 Lung.
Conclusion
FEV1 and DLCO both decline after thoracic radiotherapy,
and DLCO decline is predictable based on a well-
performing (adjusted R-square=0.71) linear-regression
model including the V5-lung. Limiting post-radiotherapy
DLCO decline would require dramatic reduction of low
lung dose, which might only be achievable using protons.
PO-0670 CPAP ventilation might allow better sparing of
normal lung tissue during lung cancer radiotherapy
D. Di Perri
1,2
, A. Colot
2
, A. Barragan
1
, G. Janssens
3
, V.
Lacroix
4
, P. Matte
5
, K. Souris
1
, X. Geets
1,2
1
Université catholique de Louvain, Center of Molecular
Imaging Radiotherapy and Oncology MIRO Institut de
Recherche Expérimentale et Clinique IREC, Brussels,
Belgium
2
Cliniques universitaires Saint-Luc, Department of
Radiation Oncology, Brussels, Belgium
3
Ion Beam Applications, Louvain-La-Neuve, Belgium
4
Cliniques universitaires Saint-Luc, Department of
Cardiovascular and Thoracic Surgery, Brussels, Belgium
5
Cliniques universitaires Saint-Luc, Cardiovascular
Intensive Care, Brussels, Belgium
Purpose or Objective
Lung toxicity is a major dose-limiting factor in lung cancer
radiation therapy (RT). By increasing lung volume,
continuous positive airway pressure (CPAP) ventilation
during treatment might allow better sparing of the normal
lung parenchyma. However, CPAP might also influence
respiration-induced tumour motion amplitude and/or
tumour position reproducibility. In this study, taking stage
I lung cancer patients as a model, we evaluate the effect
of CPAP ventilation on lung volume, tumour motion
amplitude, and tumour position reproducibility.
Material and Methods
Stage I lung cancer patients referred for stereotactic body
radiation therapy underwent two 4D-CT scans (with and
without CPAP) at two time-points: during the treatment
preparation session (T0) and the first day of treatment
(T1), resulting in four 4D-CT scans per patient (noCPAP
T0
,
CPAP
T0
, noCPAP
T1
, and CPAP
T1
). All images were
reconstructed in their time-averaged midposition (MidP)
for subsequent analysis. Gross tumour volumes and lungs
were delineated on each MidP scan.