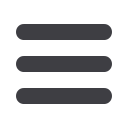

S872
ESTRO 36 2017
_______________________________________________________________________________________________
The junction in case of pat3 was covered by the 90%
isodose (except near the skin). The D99-value shows that
this treatment plan was cold compared to the other plans.
Conclusion
Measures are needed to prevent the occurrence of
extreme hot and cold spots in the junction due to DIBH
variation: This modified technique provides a damping
effect on the occurrence of dose extremes.
To create an extra buffer against underdosage, D99 of the
CTV at the junction should be high enough, eg 95%.
The technique presented is well tolerated by the patient.
EP-1631
Reproducibility of DIBH tecnique guided by an
optical system: the florence usl experience
S. Russo
1
, F. Rossi
2
, G. Stoppa
2
, L. Paoletti
2
, S. Fondelli
2
,
R. Barca
2
, P. Alpi
2
, B. Grilli Leonulli
2
, S. Pini
1
, M.
Esposito
1
, A. Ghirelli
1
, L. Cunti
2
, L. Isgrò
2
, M. Verdiani
2
,
P. Bastiani
2
1
Azienda USL Toscana Centro, Medical Physics Unit,
Florence, Italy
2
Azienda USL Toscana Centro, Radiotherapy Physics Unit,
Florence, Italy
Purpose or Objective
Aim of this work was to evaluate interfraction and
intrafraction reproducibility of a
deep inspiration breath-
hold (DIBH) tecnique based on optical surface tracking
technologies for selected patients undergoing adjuvant RT
for left-sided breast cancer.
Material and Methods
30 patients that underwent left side adjuvant
radiotherapy were included in this study. Prospective
gating CT imaging was performed by Sentinel™ (C-RAD
Positioning AB, Sweden) laser scanner system and a
Siemens BrightSpeed CT scanner. Base line level and
gating window amplitude of the respiratory signal was
established during CT simulation procedure. Gated
treatments delivery was supported by the Catalyst™
system (C-RAD Positioning AB, Sweden) connected with an
Elekta Synergy linear accelerator (Elekta AB, Sweden) via
the Elekta Response™ Interface. The treatment beam was
turned on only when the patient signal is within the
previously established gating window. Visual coaching
through video goggles were provided to help the patient
following the optimal breathing pattern. Treatments were
performed in DIBH with 3D conformal tangential beams
for 50 Gy median dose to the whole breast in 25 fractions.
The reproducibility of the DIBH during treatment was
monitored by comparing the reference CT surface with the
3D surfaces captured by Catalyst
TM
system during BH
before and after treatment delivery. Interfraction and
intra-fraction variability were quantified in mean and SD
displacements in traslation (Lat, Long, Vert) and rotations
(Rot, Roll, Pitch) in the isocenter position between the
reference and the live surface over all the treatment
fractions of the enrolled patients.
Results
Inter-fraction variability before treatment delivery was
extremely reduced: the group mean translational and
rotational errors were respectively lower than 0.4 mm
and 0.7° in all directions. After treatment delivery the
group mean shift was lower than 2 mm in all direction and
no difference in rotations was observed.
Intra-fraction variability was <2.1 mm in translations and
<1° in rotations. The cumulative distribution of
interfraction mean shift during BH before (BT) and after
(AT) treatment delivery for the patients undergoing BH
treatment is shown in figure. Separate contributions from
Lateral, Longitudinal,and Vertical direction were
reported.
Conclusion
In our experience DIBH procedure guided
by optical
systems for left breast irradiation is a reproducible and
stable tecnique with a a limited inter-fraction and intra-
fraction DIBH
variability.
EP-1632 A motion monitoring and processing system
based on computer vision: prototype and proof of
principle
N. Leduc
1
, V. Atallah
2
, A. Petit
1
, S. Belhomme
1
, V. Vinh-
Hung
3
, P. Sargos
1
1
Institut Bergonié, Radiation Oncology, Bordeaux, France
2
University Hospital of Bordeaux, Radiation Oncology,
Bordeaux, France
3
University Hospital of Martinique, Radiation Oncology,
Fort-de-France, France
Purpose or Objective
Monitoring and controlling respiratory motion is a
challenge for the accuracy and safety of therapeutic
irradiation of thoracic tumors. Systems based on the
monitoring of internal or external surrogates have been
developed but remain costly and high-maintenance. We
describe here the development and validation of
Madibreast, an in-house-made respiratory monitoring and
processing device based on optical tracking of external
markers.
Material and Methods
We designed an optical apparatus to ensure real-time
submillimetric image resolution at 4 m. Using code
libraries based on OpenCv, we optically tracked high-
contrast markers set on patients' breasts. Validation of
spatial and time accuracy was performed on a mechanical
phantom and on human breast. A simple graphical
interface allowed the user to vizualise in real-time the in-
room motion of the markers during the session.
Results
Madibreast was able to track motion of markers up to a 5
cm/s speed, at a frame rate of 30 fps, with submillimetric
accuracy on mechanical phantom and human breasts.
Latency remained below 100 ms. Concomitant monitoring
of three different locations on the breast showed
discrepancies in axial motion up to 4 mm for deep-
breathing patterns. Figure 1 displays an example of user