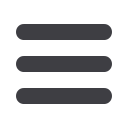

S932
ESTRO 36 2017
_______________________________________________________________________________________________
of agreement was noted for all tests (Table 1), of which
for geometric accuracy (inside length=0.4mm;
diameter=1.0mm), slice thickness accuracy (T1=0.3mm;
T2=0.5mm), slice position accuracy (T1 slice 1=1.4mm; T1
slice 11=0.5mm; T2 slice 1=0.9mm; T2 slice 11=0.6mm),
intensity uniformity (T1=0.0%; T2=0.1%), percent-signal
ghosting (0.0003) and low-contrast object detectability
(T1=2.9; T2=3.4) were all much smaller than the
corresponding ACR criterion. As illustrated in Fig. 1, all
data points fell within the 95% limit of agreement except
for diameter accuracy, for which 3 out of 54 (~5.6%) data
points fell outside the 95% limit of agreement.
Furthermore, small measurement bias close to zero was
also obtained for most tests. In terms of ICC, excellent
inter-observer agreement (ICC>0.75) was achieved in
geometric accuracy (ICC>0.99), spatial resolution (ICC =
1), slice position accuracy (ICC = 0.81), image intensity
uniformity (ICC = 0.80), percent ghosting ratio (ICC = 0.85)
and low-contrast object detectability (ICC = 0.89). A fair
inter-observer agreement was seen in the slice thickness
accuracy (ICC = 0.42). Based on both BA-analysis and ICC,
excellent inter-observer agreement could be achieved in
the ACR MRI phantom test under RT-setting.
Conclusion
Our results showed that ACR MRI phantom test under RT-
setting was highly reproducible and subject very little to
inter-observer
disagreement.
EP-1723 Optimisation of an Elekta XVI (R.5.0.2)
system for clinical protocols – image quality vs dose.
D. Oborska-Kumaszynska
1
, D. Northover
1
1
Royal Wolverhampton NHS Trust, MPCE Department,
Wolverhampton, United Kingdom
Purpose or Objective
The use of CBCT in radiotherapy has significantly
increased in recent times, which has led to an increase in
the concomitant dose received by some patients. Often,
the generic preset protocols provided by the
manufacturers are not optimised for a particular
department. This work aimed to optimise image quality
and dose for CBCT clinical protocols using an Elekta XVI
(R.5.0.2) machine for all clinically relevant treatment
sites.
Material and Methods
The Elekta XVI system was fully calibrated and Acceptance
Tests (AT) were performed for all FOVs before the
optimisation procedure. Three different phantoms were
used to complete the optimisation: CATPHAN 600, Phillips
WEP Phantom Set (PWEPPS) (5 circular objects 15, 20,
26.5, 36.5, 50cm diameter – Fig.1.) and Rando phantom
(RP). The optimisation methodology was designed to
assess dose vs the following image quality parameters:
spatial resolution (SR), uniformity (UN), contrast (CON),
CNR, SD, SNR and artefacts. These parameters were
evaluated as absolute values and compared to the
“standard” image results. These “standard” images were
taken for AT presets. The optimisation process was
performed by setting the exposure parameters: mA per
frame, ms per frame and gantry start/stop angles. The
first step involved taking CATPHAN images using varying
mA and ms settings. SR, UN, CON, CNR, SD and SNR values
were recorded. Final mA and ms settings were chosen
based on SNR and UN results, and were no worse than 20%
and 5% respectively in relation to the “standard image”.
Images were also compared using the same mAs but
different mA and ms values. The second step involved
taking PWEPPS images using the final mA and ms settings
for each protocol. SNR and UN were evaluated for
phantom diameters relevant to the treatment site in
question. The RP was used to assess image quality for the
finalised clinical protocols
.
Results
The optimisation process resulted in better image quality
in relation to the original presets and “standard images”.
Dose was reduced by a factor ranging from 2-4 times. For
a given mAs, superior image quality was seen for a higher
mA and lower ms, indicating that the detector response
was better for a higher dose rate. Saturation artefacts
(Fig.2) were visible for 64mA and 10ms when the images
included the intersection between the test object and air.
The worse UN was seen for LFOV. This was affected by
“cutting” from the reconstruction 40 pixel rows at the
edge of the panel. It was done because the bad pixel map
correction algorithm could not effectively correct the bad
pixels. Additionally, for 2D kV images, bad pixel artefacts
were visible using the TOR18FDG phantom. The kV
detector panel was replaced and the new one was
calibrated to get similar gains so the optimisation process