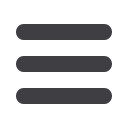

141
7
basal portion of the cochlea. However, psychophysical SOE measurements did not exhibit differences along
the array [Cohen et al., 2004;Nelson et al., 2008]. The advantage of measuring SOE with eCAPs is that
the measurements provide information about the electrode-neural interface and whether psychoacoustic
measures are also influenced by central auditory pathways. However, no significant correlation between
eCAP-measured SOE and speech perception has been demonstrated [Hughes and Stille, 2010;van der Beek
et al., 2012]
[Chapter 4]
. Furthermore, eCAP SOE measures are strongly influenced by various parameters
[van der Beek et al., 2012;Hughes and Stille, 2010]
[Chapter 4]
. This result indicates that care should be
taken when considering statements about eCAP measurements of the SOE in the cochlea. Additionally,
this finding is consistent with the fact that eCAP thresholds are not sufficiently correlated with the clinical
stimulation levels required for fully automated fitting [Botros and Psarros, 2010]. In short, eCAPs can
provide information about the electrode-neural interface, although more research is needed to link the data
with clinical outcomes. As Chapter 4 shows, the parameters that influence eCAP measurements must be
determined before eCAPs can be used effectively to analyze or predict clinical outcomes.
Measuring outcomes using clinical stimulation levels
In addition to eCAPs and speech perception scores, stimulation levels provide information about the
effectiveness of the electrode-neural interface, especially how effectively the cochlear implants promote
sound perception. Moreover, it is likely that thresholds and maximum levels are less influenced by central
effects than speech perception scores are. In addition, T-levels can measure the effects of the electrode array
design in different areas of the cochlea. The threshold levels for electrical stimulation are theoretically a good
measure of the stimulation’s effectiveness. T-levels exhibit a positive correlation with speech perception [van
der Beek et al., 2015b]
[Chapter 5]
; however, only a few studies that included large groups of patients
present T-level data [Vargas et al., 2012;van der Beek F.B. et al., 2014;van der Beek et al., 2015a]
[Chapter
5,6]
. It is difficult to compare patient data given the considerable interpatient variability in levels, especially
when stimulation levels are expressed as linear current units, which is the default setting in Advanced
Bionics software (Advanced Bionics Corp., Sylmar, CA, USA). Moreover, level data comparisons among
cochlear implants produced by different manufacturers are hindered by the use of different scales for
stimulation levels. For example, manufacturer Cochlear Corp. (Lane Cove, Australia) uses current levels,
which are measured with a logarithmic scale. Logarithmic scales can reveal intrapatient differences even in
cases of significant overall level variation. Stimulus levels can be recalculated to demonstrate intrapatient
effects by converting the linear scale to dB [Pfingst et al., 2004;van der Beek F.B. et al., 2014]
[Chapter 5]
or by normalizing the data [Vargas et al., 2012]. Additionally, few studies contain large collections of T-level
data because in many centers, T-levels are not measured clinically for each electrode contact. This lack exists
because studies have reported that T-level settings do not have a significant effect on speech perception
[Boyd, 2006]. Although these studies contained only a limited number of patients, and other studies have
demonstrated the value of establishing T-levels [Spahr and Dorman, 2005;Holden et al., 2011], T-level
measurement in many centers is limited to a few electrodes, and interpolation is used to expedite fitting.
Alternatively, T- levels may not be measured and instead are set at 10% of the M-levels [Baudhuin et