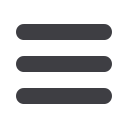

668
S
chneider
&
A
ndersen
:
J
ournal of
AOAC I
nternational
V
ol
. 98, N
o
. 3, 2015
would not produce statistically relevant determinations of MDL
and LOQ.
The method accuracy generally yielded analyte trueness
greater than 90%. In order to determine the extent of correction
provided by the combination of extracted matrix calibrants
and internal standard correction, participants were requested
to concurrently analyze a set of solvent calibrants and a single
post-extraction fortified matrix calibrant (QC, 1.0 µg/kg) along
with the study samples. Internal standard corrected peak area
ratios for the 0.90 ng/g fortified sample results generated by
10 laboratories were converted to concentrations using the three
different methods of calibration: (
1
) extracted matrix calibration
curves (the quantitative method used in this collaborative study),
(
2
) single point calibration against the post-extraction fortified
matrix QC calibrant, and (
3
) solvent calibration curve (Table 5).
For the single point QC calibration method, the 0.90 µg/kg
fortified samples were normalized relative to the 1.0 µg/kg QC
calibrant.
There was generally good agreement among the three
calibration methods for all analytes except for BG, where
the post-extraction fortified QC matrix calibrant and solvent
calibration curve overpredicted the concentration of the residue
level and often led to poorer repeatability. Differences in method
performance betweenBGandMG-D5may account for variations
in the analyte accuracy for BG. BG quantification was studied
in greater detail with respect to internal standard correction and
matrix effects in a complementary single-laboratory validation
study of this method (18). While the post-extraction fortified
QC matrix calibrant produced the highest calculated recoveries
generated by the three methods, there was generally little
difference between the average recoveries generated by the
extracted matrix calibration curves and the solvent calibration
curves for the analyteswithmatched isotopically-labeled internal
standards (MG, LMG, CV, and LCV). The variance in analyte
recovery using solvent calibration curves was also generally the
largest. A more extensive investigation of this phenomenon has
been reported (18). In that work, method accuracy determined
from data collected by a single laboratory for MG, LMG, CV,
and LCV was found to be generally comparable regardless of
which calibration method was used, as long as internal standard
correction was applied. The post-extraction fortified calibrant
trueness for BG matched the collaborative study results with
enhanced recoveries (129–163%); however, in the single-
laboratory validation, very low recoveries were found for
BG using solvent based calibrants (0–64%). From the results
of both studies, and the procedure described by First Action
2012.25
(8), it is clear that acceptable method trueness for all
analytes is achieved only when extracted matrix calibrants with
internal standard correction are used for quantitative analysis.
Qualitative Results
Analyte identification was achieved by comparison of
peak area ratios of the qualitative:quantification product ion
transitions of test samples to the average value of the ratios
obtained from extracted calibrant samples (0.25–5 µg/L). These
results are summarized in Table 6. Acceptability criteria for
both the EU (±20–50%, based on ratio found; 10) and the FDA
(±10% absolute; 11) were applied to the data, and the results
were compared. It is interesting to note that while there were
individual cases where one or the other approach provided higher
identification percentages, on the whole, the two approaches
provided comparable results. Evaluation of retention times for
identification revealed two laboratories that had some difficulty
in meeting the stricter EU standard (±2.5%) on a total of six or
13 samples, respectively. All samples, however, met the FDA
retention time standard (±5%).
In general, identification was successful for the overwhelming
majority of samples. Blank samples did occasionally meet
identification criteria, particularly for CV and LCV, as
evidenced by the higher percentage of identifications listed
for those blank samples (Table 6). Although these samples did
meet the requirement of having signals greater than three times
the instrument noise, the calculated concentrations for most
blank samples was below 0.05 µg/kg. Thirteen of the 84 blank
samples met identification criteria and had a calculated analyte
concentration >0.05 µg/kg; calculated concentrations for those
individual blank samples are reported in Table 6. Five of those
blank samples have analytes with concentrations at or above
the MDL for the particular analyte/matrix pair: 0.08 μg/kg
LMG in salmon, 0.14 μg/kg CV in salmon, 0.30 μg/kg CV
in shrimp, and 0.12 and 0.13 μg/kg LCV in catfish. None of
the blank samples have analyte concentrations that exceed the
CCα for the analyte/matrix pair. In general, concentrations of
the identified analytes in the blank samples are well below the
1 µg/kg level of concern. True false-positive samples may be a
result of instrument carryover or trace contamination with ink
from commonly used laboratory marking pens. For best results,
it is advisable to inject water samples between test samples to
identify and minimize interference (8), and to avoid the use of
laboratory marking pens when labeling samples.
Use for Screening
The authors of the first action method proposed that this
method could be used as a screening method by estimating
the concentration of residues in an unknown sample by
comparison to a single point extracted matrix calibrant spiked
at 0.5 µg/kg. In that analytical strategy, unknown samples that
yielded corrected peak areas greater than those generated for
the 0.5 matrix calibrant would require a secondary analysis
with a full calibration curve (8). From the results of the
14 participating laboratories, peak area data (internal standard
corrected) for each 0.5 µg/kg extracted matrix calibrant was
tabulated and compared to the appropriate (matching analyte
and matrix) corrected peak area for the 10 blinded unknown
samples analyzed by each laboratory. The percentage of blinded
samples that yielded peak areas greater than the peak area of
the 0.5 µg/kg calibrant is summarized in Table 7. None of
the negative control samples yielded peak areas greater than
the 0.5 µg/kg calibrant, and all of the 1.75 µg/kg fortified
samples had responses greater than the 0.5 µg/kg calibrant. For
the 0.42 µg/kg fortified samples, 14% (58 of the 420 analyte
measurements; five analytes × three matrixes × duplicate
samples × 14 laboratories) yielded peak areas greater than the
0.5 µg/kg calibrant. Of these, 22 of the analyte measurements
(5%) yielded peak areas >20% of the 0.5 µg/kg calibrant,
corresponding to concentrations of 0.62 to 1.14 µg/kg based
on the single calibrant estimation. Table 7 highlights the screen
results/analyte; however, it should be noted that one sample often
yielded incorrect screen results for more than one residue. For
example, catfish at the 0.42 µg/kg level yielded several samples