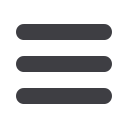

ESTRO 35 2016 S3
______________________________________________________________________________________________________
radiotherapy (IGRT), has highlighted deficiencies in target
delineations based on CT. Several studies have shown large
variability in target definitions based on CT, for multiple
treatment sites. To address this issue, magnetic resonance
imaging (MRI) has made its way into the clinical routine at
modern radiotherapy departments over the last years. This,
however, has presented several new problems that need to
be solved.
The traditional method of including MR information in the
radiotherapy process is as a complement to the CT. To
accomplish this in an integrated and accurate fashion, the
images must be placed in a common coordinate system
through image registration. This process in itself introduces
new uncertainties into the treatment chain, which must be
quantified and minimized. Another method of using MR
information is to base the entire treatment on MR and
exclude the CT altogether. This alleviates uncertainties that
stem from the image registration process, but introduces
another set of problems. To perform accurate dose
calculations, heterogeneity corrections based on CT data
have been the clinical standard for many years. MR data does
not provide information that can be used for such
corrections; however, much research effort has been
invested in creating valid photon attenuation maps from MR
data over the last years.
Whatever method employed, MR for radiotherapy purposes
also imposes practical issues that need to be addressed. The
patient needs to be positioned in the same way that will be
employed during the radiotherapy itself. This includes a flat
table top and immobilization devices such as cast masks and
tilted boards, which may not be MR compatible. For example,
many radiotherapy fixation devices can contain metal parts
such as nuts and bolts, which cannot be used in the MR.
Plastic replacements must be used instead. Also, the
standard MR coils will often not accommodate the
immobilized patient, which forces MR adopters to acquire
special coils or coil holders for flexible coils to be able to
scan the patient in the radiotherapy treatment position.
MR images do not have the same geometric integrity as CT,
which is an issue in the radiotherapy setting. The image
distortions can come from the machine itself or from the
patient that is in the machine. Machine specific distortions
are caused by inhomogeneity in the main magnetic field or
gradient non-linearity. Patient specific distortions are mostly
caused by susceptibility effects. The machine specific
distortions can be measured, modelled and corrected for to a
certain extent, while patient specific distortions often needs
to be handled by choosing imaging parameters wisely.
In the end, the images acquired from the MR scanner must be
of sufficient quality to allow physicians to base the
radiotherapy treatment on them. MR for radiotherapy has a
different set of demands on the images than their diagnostic
counterparts, for example slice thickness and gap, as well as
other parameters. Also, the vast variety of MR contrasts may
be an initial obstacle for radiotherapy oncologists. Many
studies have shown differences in target definitions based on
CT and MR images, and the effects of these changes in target
volumes have not yet been studied in clinical trials.
Teaching Lecture: Patient specific quality assurance in
proton therapy
SP-0007
Patient specific quality assurance in proton therapy
R. Amos
1
University College London Hospitals NHS Foundation Trust,
Department of Radiotherapy Physics, London, United
Kingdom
1
Interest in proton therapy continues to grow worldwide, yet
access to proton therapy facilities remains relatively low
compared to those offering conventional radiotherapy. As a
consequence, pressure exists to maximize patient throughput
in each facility. Most facilities operate 24 hours per day, 7
days per week to meet the demands of the clinical load and
to complete machine maintenance, routine quality
assurance, and patient specific quality assurance. With the
advent of advanced delivery techniques such as pencil beam
scanning, the complexity of patient specific quality assurance
is increasing. However, there is a need to improve efficiency
of these tests whilst maintaining accuracy.
This presentation will summarize contemporary patient
specific quality assurance practice for both passive scattering
and pencil beam scanning proton therapy, and describe off-
line tests that potentially enable improved efficiency.
Teaching Lecture: Balancing toxicity and disease control in
the evolution of radiotherapy technology
SP-0008
Balancing toxicity and disease control in the evolution of
radiotherapy technology
B. O'Sullivan
1
Princess Margaret Cancer Centre, Toronto, Canada
1
, S. Huang
2
2
Princess Margaret Cancer Centre/University of Toronto,
Radiation Oncology, Toronto, Canada
Radiotherapy (RT) is an effective option for treatment of
many cancers. It offers organ and functional preservation and
enhances surgical outcomes when administered pre-
operatively or post-operatively, and for some diseases, such
as nasopharyngeal cancer, it is often the only curative
option. Disease control is generally of paramount importance
to most patients during the urgent point of decision-making
following diagnosis. However toxicity will almost certainly
emerge as being just as relevant in the aftermath of
treatment and in the subsequent follow-up period. In
essence, when a patient dies of toxicity or treatment-related
complications, it is just as tragic as dying of disease. The
long-term result of RTOG 9111 and 9501 suggest that
treatment -related deaths are blunting originally observed
difference in cancer-related outcome. The recent RTOG 0617
trial was designed to test whether a higher RT dose (74 Gy vs
60 Gy) +/- cetuximab could confer a survival benefit but
showed an unexpected therapeutic “disadvantage” with
higher RT dose attributable to significant acute and late
toxicities. These findings highlight the importance of
balancing toxicity and disease control to optimize
therapeutic gain. Several strategies have been employed to
mitigate toxicities, such as respecting the biology of
radiation injury by altered dose fractionation (typically using
smaller than conventional fractions), or optimising
radiotherapy technical delivery to reduce dose to vulnerable
anatomy. Implementing novel RT technologies need to be
closely monitored to prove clinical benefit. Historical lessons
have shown that putative benefits may not always transfer to
real clinical advantages since many unforeseen factors may
modify potential anticipated gains. While modern RT
technologies, such as IMRT-IGRT, adaptive, and IMPT provide
opportunities to reduce RT late toxicity by providing more
conformal dose distribution to spatially avoid normal tissue,
the steps to achieve this are complex. One needs to
appreciate many diverse factors. These include radiobiology
of normal tissue (dose/constraints), optimal imaging quality
and registration, systematic quality control involving “target”
delineation to delivery, and knowledge of a variety of
inherent pitfalls in the process(e.g. poor delineation, dose
dumping, erratic planning, tumor or normal tissue
deformation, and set up uncertainties that may emerge
throughout the treatment course). For example, beam path
toxicities have been reported due to “dose dumping” from
parotid-sparing IMRT in head and neck cancer. Increased
local failure has been observed when delivering tight margin
carotid-sparing partial organ irradiation for T2 glottic cancer
using vertebrae rather than laryngeal soft tissue as the image
guidance surrogate. Adaptive radiotherapy appears to be
feasible in some situations but the therapeutic advantages
are yet to be proven and may be tedious and inefficient
under the current technical configurations of many
departments. Also, while intensity-modulated proton therapy
(IMPT) is an attractive emerging approach that is probably
able to spare normal tissue, indications and clinical benefit
are also largely unproven at this time. The path to
implementing these approaches will require rigorous