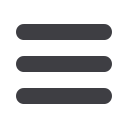

S260
ESTRO 35 2016
_____________________________________________________________________________________________________
7
Medisch Spectrum Twente, Radiotherapy, Enschede, The
Netherlands
8
VSL, VSL, Delft, The Netherlands
9
University Medical Centre Utrecht, Department of
Radiotherapyy, Utrecht, The Netherlands
Purpose or Objective:
To independently validate patient-
specific quality assurance (QA) methods, clinically used in the
Netherlands, for IMRT and VMAT plans using the same set of
treatment plans for all institutes.
Material and Methods:
A set of treatment plans was devised:
simple and more complex IMRT/VMAT and a stereotactic
VMAT plan, all 6MV for both Varian and Elekta linacs. Ten
plans were used for Varian linacs (5 for True Beam and 5 for
Clinac) and 9 for Elekta linac(4 for MLCi and 5 for Agility).
The plans were imported in the participating institute’s
treatment planning system for dose computation on the CT
scan of the audit phantom (provided by the audit team
together with the plans). Additionally, 10x10 cm2 fields were
made and computed on both phantoms. Next, the audit team
performed measurements using the audit equipment. All 21
Dutch radiotherapy institutes were audited. The
measurements were performed using an ionization chamber
(PinPoint, PTW), Gafchromic EBT3 film and a 2D ionization
chamber array, all in an octagonal phantom (Octavius, PTW).
Differences between the measured and computed dose
distribution were investigated using a global gamma analysis
with a 5%/1mm criterion for the stereotactic VMAT plan and
3%/3mm for the other plans with a 95% pass rate tolerance.
Additionally, the participating centres performed QA
measurements of the same treatment plans according to
their local protocol and equipment.
Results:
The average difference between the point
measurement, at the centre of the phantom, and the planned
dose is below 1% (range: (-4.0 – +2.0)%) independently on the
plan type (table 1).
As shown in figure 1 the average pass rate obtained from the
array measurements is in good agreement (average
difference: (0.4 ± 1.0)%) with the average pass rate of the QA
measurements provided by the participating institutes
performed with their equipment for all the plans except for
the simple VMAT plan.
For the latter, the pass rate obtained with the Octavius is
influenced by the sensitivity variation of the array as a
function of gantry angle. Seven institutes out of 21 had plans
that failed the audit gamma analysis pass rate tolerance of
95% while the institute’s QA outcome was within tolerance (1
institute two plans, 6 institutes one plan). The film
measurement results are still under investigation and
therefore not presented in this abstract.
Conclusion:
The results demonstrate that such a national QA
audit is feasible. The reported in-house QA results were
consistent with the audit despite differences in dosimetry
equipment and analysis methods. Of the 21 Dutch centres
audited, 67% passed the gamma analysis test for all the plans
measured with a 2D-array by the audit team showing
acceptable implementation of IMRT and VMAT delivery.
OC-0546
The development of proton-beam grid therapy (PBGT)
T. Henry
1
Stockholm University, Department of Medical Radiation
Physics, Stockholm, Sweden
1
, A. Valdman
2
, A. Siegbahn
1
2
Karolinska Institutet, Department of Oncology and
Pathology, Stockholm, Sweden
Purpose or Objective:
Radiotherapy with grids has previously
been carried out with photon beams. The grid method is used
as an attempt to exploit the clinical finding that normal
tissue can tolerate higher doses as the irradiated volumes
become smaller. In this work we investigated the possibilities
to perform proton-beam grid therapy (PBGT) with millimeter-
wide proton beams by performing Monte Carlo simulations of
dose distributions produced by such grids. We also prepared
proton-grid treatment plans with a TPS, using real patient
data and beam settings available at modern proton therapy
centers.
Material and Methods:
Monte Carlo calculations were
performed using TOPAS version 1.2.p2 in a 20x20x20 cm3
water tank. The beam grids (each containing 4x4 proton
beams arranged in a square matrix) were aimed towards a
cubic target at the tank center. A total of 2x2 opposing grid
angles were used. The target was cross-fired in an interlaced
manner. A beam-size study was carried out to find a suitable
elemental beam size regarding beam thinness, peak-to-
entrance dose ratio and lateral penumbra along the beam
path. Dose distributions inside and outside of the target were
calculated for beam center-to-center (c-t-c) separations
inside the grids of 6, 8 and 10 mm.
The TPS study was performed with Varian Eclipse. We re-
planned two patients (one liver cancer and one rectal cancer
patient) already treated in the hospital with photon therapy
with the suggested PBGT. The IMPT method was used to
prepare these plans. The plan objectives were set to create a
homogeneous dose inside the target.
Results:
A beam size of 3 mm (FWHM) at the tank surface
was found suitable from a dosimetric point of view for the
further studies. By interlacing simulated beam grids from
several directions, a cubic and nearly homogeneous dose
distribution could be achieved in the target (see Figure 1).
The c-t-c distance was found to have a significant impact on
the valley dose outside of the target and on the homogeneity
of the target dose. In the TPS study, a rather uniform dose
distribution could be obtained inside of the contoured PTV
while preserving the grid pattern of the dose distribution
outside of it. The latter finding could be important for tissue
repair and recovery.