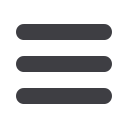

ESTRO 35 2016 S35
______________________________________________________________________________________________________
phantom, both parallel and perpendicular to the magnetic
field, and in water (waterproof chambers only). The
influence of the distribution of air around the chambers in
the SW phantom was measured by displacing the chamber in
the insert using a paper shim, approximately 1 mm thick,
positioned in different orientations between the chamber
casing and the insert.
Results:
The responses of the three waterproof chambers
measured on the MR-linac increased by 0.6% to 1.3% when
the air volume in the insert was filled with water. The
responses of the chambers on the Agility linac changed by
less than 0.3%. The angular dependence ranged from 0.9% to
2.2% in solid water on the MR-linac, but was less than 0.5% in
water on the MR-linac and less than 0.3% in SW on the Agility
linac. An example of the angular dependence of a chamber is
shown in Figure 1.
Changing the distribution of air around the chamber induced
changes of the chamber response in a magnetic field of up to
1.1%, but the change in chamber response on the Agility was
less than 0.3%.
Conclusion:
The interaction between the magnetic field and
secondary electrons in the air volume around the chamber
reduces the charge collected by between 0.6 and 1.3%. The
large angular dependence of ion chambers measured in SW in
a magnetic field appears to arise from a change of air
distribution as the chamber is moved within the insert, rather
than an intrinsic isotropy of the chamber sensitivity to
radiation. It is therefore recommended that reference
dosimetry measurements on the MR-linac be performed only
in water, rather than in SW phantoms.
OC-0076
Towards MR-Linac dosimetry: B-field effects on ion
chamber measurements in a Co-60 beam
J. Agnew
1
The Christie NHS Foundation Trust, CMPE, Manchester,
United Kingdom
1
, G. Budgell
1
, S. Duane
2
, F. O'Grady
1
, R. Young
1
2
National Physical Laboratory, Radiation Dosimetry Group,
Teddington, United Kingdom
Purpose or Objective:
To quantify the effect of small air
gaps at known positions on ionisation chamber (IC)
measurements in the presence of a strong magnetic (B-)field,
and to characterise the response of ICs over a range of B-
field strengths in the absence of air gaps.
Material and Methods:
The ratio of responses of four
commercially available ICs was measured in a Co-60 beam
with and without a 1.5T B-field (
M
1.5T
/
M
0T
) using a GMW
electromagnet unit and a 5cm pole gap. Measurements were
made in custom-built Perspex phantoms with the chamber,
beam and B-field all orthogonal. The measurements were
repeated with the phantoms at each cardinal angle (rotated
about the long axis of the ICs). The phantoms were designed
to be symmetric under rotation about this axis except for a
shallow 90° section next to the sensitive volume of the ICs.
The measurements were repeated with the air gap removed
by introducing water to the phantom cavity. For the PTW
30013 chamber further measurements were performed after
introducing a small (approximately 30 mm
3
) bubble into the
recess when the cavity was otherwise filled with water,
which was made possible by the novel phantom design. The
measurements in water were repeated with additional build-
up material and in multiple phantoms at a single phantom
orientation.
Measurements were also taken to characterise the ratio of
responses for five ICs over a range of B-field strengths (0 – 2T
in 0.25T increments).
Results:
For all 4 ICs in the rotating setup, the response
varied consistently with the position of the recess when the
air gap was present, with the lowest value of
M
1.5T
/
M
0T
obtained when the recess was upstream of the IC. The
maximum peak-to-peak (PTP) variation was 8.8%, obtained
for the PTW 31006 ‘Pinpoint’ IC, and the minimum was 1.1%,
obtained for the Exradin A1SL IC. This variation all but
disappeared (maximum PTP variation 0.7%, seen for PTW
31010 IC) when the air gap was removed. A large (3.9%) PTP
variation was observed for the PTW 30013 when an air bubble
was inserted into an otherwise airless setup (0.2% variation
without air gap).
Conclusion:
Small air gaps are responsible for large variations
in IC response in the presence of a magnetic field. These
variations can be eliminated by introducing water into the
cavity, but even small bubbles will cause large variations in
the response. Further, IC response in the presence of a 1.5T
B-field is insensitive to changes in depth and scatter
conditions of the phantoms investigated here. Each IC has
different
M
/
M
0T
response across the range of B-field strength
0 – 2T.
OC-0077
Dual energy CT proton stopping power ratio calibration:
Validation with animal tissues
Y. Xie
1
University of Pennsylvania, Department of Radiation
Oncology, Philadelphia, USA
1
, L. Yin
1
, C. Ainsley
1
, J. McDonough
1
, T. Solberg
1
, A.
Lin
1
, B.K. Teo
1
Purpose or Objective:
One main source of uncertainty in
proton therapy is the conversion of Hounsfield Unit (HU) to