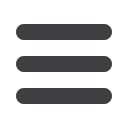

are generally even less stable with
tolerances of ±100ppm.
Classical RF analog transceivers
and receivers are typically built with
phase-coincidence demodulators.
They will generally employ either
an external discriminator circuit or
an integrated FSK demodulator. As
a result of the analog demodulation
principle, these products offer a
carrier frequency acceptance range
of up to ±100kHz.
If we consider the carrier frequency
of an IoT transmitter node of
868.3MHz based upon a low-cost
crystal reference that has a tolerance
of ±50ppm, the center frequency
of the node can have a spread of
about ±43kHz. This value may
already exceed the FSK deviation,
which is an essential modulation
parameter. Typical allowable FSK
deviation values for IoT sensor node
applications are between ±10kHz to
±50kHz. Nevertheless, RF products
with analog demodulators can cope
with carrier frequency spreads that
are larger than the FSK deviation
due to their wide carrier frequency
acceptance.
Modern highly integrated RF
products perform the demodulation,
and many other necessary signal
conditioning operations, in the
digital domain. This is possible due
to modern semiconductor processes
that are based on small geometries,
thus leading to very compact IC
designs. However, due to their
digital nature, most modern RF
transceivers exhibit relatively small
carrier frequency acceptance ranges,
compared to their legacy analog
counterparts. Therefore, receiving
a signal from an IoT sensor node
can be a challenge for a digital RF
receiver if the sensor node exhibits
a poor frequency accuracy because
of a wide tolerance crystal.
GHz often refers to one of the ISM
bands, for example at 433.92MHz or
868.3MHz.
A 2.4GHz based system offers a
relatively high data throughput,
often it is in the order of several
Megabits-per-second (Mbps) for Wi-
Fi and substantially less at around
260 kbps for BLE. Obviously, Wi-Fi
is compatible to WLAN infrastructure
such as routers and can therefore
directly connect to the IoT. The
various incarnations of Bluetooth can
connect directly to a mobile device,
which can then, in turn, provide a
connection to the IoT / Internet. One
downside of a 2.4GHz wireless link
is its relatively short range (<10m)
due to the high propagation losses
that occur in comparison to Sub-GHz
based systems.
Sub-GHz is an ideal choice for use
if long range (up to 1km outdoors)
is important to the application or
installation. Sub-GHz provides high
levels of robustness as well as
excellent immunity against disturbing
signals through the use of narrow-
band radio channels (often around
25kHz). Furthermore, as Sub-GHz
devices typically run on proprietary
protocols, it is relatively easy to
optimize them for power efficiency
and long battery life; both essential
for battery powered or energy-
harvesting IoT remote sensors.
Fig. 2 illustrates a number of IoT
applications that benefit significantly
from Sub-GHz technology.
Carrier frequency
acceptance matters
An important element when using
wireless links for any application,
but especially IoT applications, is
the ability for the receiving nodes
to track carrier frequency deviations
of the transmitting nodes. Modern
integrated RF receivers and
transmitters use quartz crystal
technology for generating a local
reference frequency within each
device. Affordable crystals typically
exhibit frequency stabilities in
the range of ±10ppm to ±50ppm
approximately. Less integrated RF
products, which are often based on
devices such as SAW resonators,
Fig. 2: Sub-GHz applications space
IoT
Special Edition
New-Tech Magazine Europe l 59