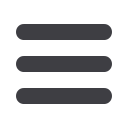

S140
ESTRO 36 2017
_______________________________________________________________________________________________
analyzed the factors associated with the difference of the
whole prostate dose between the two dosimetry. This is
the first report which evaluated those factors using 3-
Tesla MRI in which contouring and fusion are thought to be
more accurate than in 1.5-Tesla MRI.
Material and Methods
The subjects were 81 consecutive patients treated with
144 Gy of brachytherapy alone using loose I-125
radioactive seeds. For postimplant analysis, CT and MRI
scans were obtained at 1 month after implantation. CT
and 3-Tesla T2-weighted MR images were fused and
aligned on the basis of seed distribution in MRI/CT fusion-
based dosimetry. Dosimetry was computed for the whole
prostate and for the prostate divided into anterior and
posterior sectors of the base, mid-gland, and apex (Fig.
1). The volumetric and dosimetric results were compared
between MRI/CT fusion-based and CT-based dosimetry
using a paired t test. Factors associated with the absolute
value of the difference of D90 between the two dosimetry
(|D90MRI/CT - D90CT|) were analyzed by multiple
regression. P values of <0.05 were defined to be
significant.
Results
D90 (176.7 Gy vs 173.0 Gy; p = 0.003) and V100 (97.2% vs
96.5%; p = 0.013) were significantly higher in MRI/CT
fusion-based dosimetry than in CT-based dosimetry.
Prostate volume (28.5 mL vs 30.8 mL; p < 0.001) was
significantly lower in MRI/CT fusion-based dosimetry than
CT-based dosimetry. Sector analysis showed a decrease in
MRI/CT fusion D90 at the anterior base (154.9 Gy vs 166.5
Gy; p < 0.001) and the posterior apex (169.7 Gy vs 177.6
Gy; p < 0.001), and increase in MRI/CT fusion D90 in the
anterior mid-gland (195.2 Gy vs 181.7 Gy; p < 0.001), the
posterior mid-gland (196.1 Gy vs 193.9 Gy; p = 0.030), and
the anterior apex (198.7 Gy vs 175.0 Gy; p < 0.001).
|D90MRI/CT - D90CT| was largest at the anterior apex
sector among 6 sectors (27.2 Gy). On multivariate
analysis, |D90MRI/CT - D90CT| of whole prostate are
associated with |prostate volume (PV)MRI/CT - PVCT| (p
= 0.036), |D90MRI/CT - D90CT| at the posterior base
sector (p = 0.035), |D90MRI/CT - D90CT| at the anterior
mid-gland sector (p = 0.011), and |D90MRI/CT - D90CT| at
the anterior apex sector (p = 0.004) (Table 1).
Conclusion
Several postimplant dosimetric variables were
significantly different on MRI/CT fusion vs CT. The
differences between the two methods of PV, D90 at the
posterior base, anterior mid-gland, and anterior apex
sectors may greatly influence the difference of D90 of the
whole prostate.
Proffered Papers: Physics treatment verification
OC-0275 Testing an MR-compatible afterloader for MR-
based source tracking in MRI guided HDR
brachytherapy
E. Beld
1
, P.R. Seevinck
2
, J. Schuurman
3
, F. Zijlstra
2
, M.A.
Viergever
2
, J.J.W. Lagendijk
1
, M.A. Moerland
1
1
UMC Utrecht, Department of Radiotherapy, Utrecht,
The Netherlands
2
UMC Utrecht, Image Sciences Institute, Utrecht, The
Netherlands
3
Elekta NL, Veenendaal, The Netherlands
Purpose or Objective
In HDR brachytherapy, image guidance is crucial for
accurate and safe dose delivery. Accordingly, MR-guided
HDR brachytherapy is in development at our institution.
This study demonstrates the testing of a recently
developed MR-compatible afterloader, while operating
simultaneously with MR imaging, as well as an MR-based
method for real-time source position verification.
Material and Methods
Experimental set-up:
A prototype of an MR-compatible afterloader (Flexitron,
Elekta) was developed. This afterloader was made MR-
compatible by providing every part as well as the cover
with RF shielding. The source cable was replaced by a
plastic cable containing a piece of steel at its tip, serving
as a dummy source. The afterloader was placed next to
the MRI scanner and connected to a catheter positioned in
an Agar phantom (doped with MnCl2), see Fig. 1.
Afterloader management:
The afterloader was programmed to send the source (I) to
10 dwell positions, with a 10 mm step size, remaining 10 s
at each position, and (II) to 20 dwell positions, with a 5
mm step size, remaining 0.5 s at each position.
MRI acquisition:
While sending the source to its predefined dwell positions,
MR imaging was carried out on a 1.5 T MR scanner (Ingenia,
Philips) using a 2D gradient echo sequence (TR/TE 2.2/1.0
ms, slice thickness 10 mm, FOV 192x192 mm, acq. matrix
96x96, flip angle 30°, SENSE=2), scanning two orthogonal
slices interleaved with a temporal resolution of 0.114 s per
image.
HDR source localization:
The MR artifact induced by the magnetic susceptibility of
the metallic source was exploited. The artifacts (complex
data) were simulated based on the susceptibility induced
B0 field disturbance [1]. The localization was executed
offline in a post processing operation by phase-only cross
correlation [1,2], to find the translation between the
experimental image and the simulated artifact.
Results
The experiments demonstrated that the prototype MR-
compatible afterloader and the MRI scanner fully
functioned while operating simultaneously, without
influencing each other. The afterloader was able to send
the source to the predefined dwell positions when placed
next to the MRI scanner, without being attracted to or
being disturbed by the scanner. The HDR source positions
could be determined by the described localization method
(now accomplished offline), see Fig. 2. The average
distances between the determined 3D source positions for
cases (I) and (II) were 9.9±0.2 mm and 5.0±0.2 mm,
respectively. The short dynamic scan time (~0.15 s) and
the fast reconstruction/post processing (<0.15 s)
guarantee that source localization will be possible in real
time.
Conclusion
The MR-compatible afterloader developed in this study
and a commercial 1.5 T MRI scanner were demonstrated
to fully function while operating simultaneously, enabling
real-time HDR source position verification for MR-guided