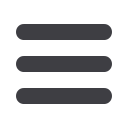

S7
ESTRO 36 2017
_______________________________________________________________________________________________
quantification of loss-risk-distributions becomes a
necessity. The term “robust optimization” is commonly
used to express that the source of the uncertainty is
directly considered during dose optimization instead of
solving a substitute problem that is construed to yield a
robust result (such as the PTV concept). The method that
currently receives most interest is the worst-case
scenario, which aims to ensure that the target volume
receives a minimum dose under a reasonable number of
displacements and range uncertainties. Although this
approach is greatly superior to the PTV concept for
particle beams, it shares the similarity that it makes no
assumptions about the frequencies with which
uncertainties occur. In other words, all scenarios are
equally likely, which is contrary to intuition (and good
practice!) and increases the price for target dose
robustness in a population. Probabilistic planning adds
the quantification of risks to robust optimization and
therefore requires assumptions about the frequencies of
uncertainties as input. A number of probabilistic
planning concepts have been proposed for photon
therapy, which all rely in some way or other on
approximations and assumptions that will not hold for
particle beams, primarily because the geometric changes
disturb the dose distributions too much. In order to keep
the problems manageable and the number of scenarios
realistically high, new methods for dose evaluation in
terms of loss-risk distributions need to be deployed. New
formulations of the optimization problem can be devised
that take advantage of these distributions. Numerical
complexity is only a secondary issue on current computer
hardware. A primary problem is the dependence on
assumptions about the frequency of uncertainties, and
the patient-individual component of these frequency
distributions. Hence, it is to be expected that reality
demands a restricted use of such approaches.
Symposium: CT imaging, new developments
SP-0022 Current status and potential of dual energy
and spectral CT
J. Andersson
1
1
Norrlands Universitetssjukhus, Department of Radiation
Sciences- Radiation Physics, Umea, Sweden
Conventionally, Computed Tomography (CT) images are
reconstructed with data that has been collected using a
single X-ray tube potential during a scan. CT images are
presented in the Hounsfield scale, which is based on linear
attenuation coefficients that have intrinsic energy
dependence.
A method for Dual Energy Computed Tomography (DECT)
imaging was first proposed in the 1970’s, where two sets
of data are collected with different radiation qualities.
These two data sets are used to draw conclusions about
the material that has been scanned by analysing the
energy dependence of the linear attenuation coefficients.
In recent years, the major CT manufacturers have adopted
DECT and several technological solutions are available on
the market, including CT scanners with two X-ray tubes,
X-ray tubes with rapid tube voltage switching and
detectors with two layers that provide an energy
separation of the incoming photon spectrum.
One of the major benefits of using DECT is that materials
can be viewed in terms of composition and mass density.
Further benefits of DECT include mitigation of specific
artefacts in the CT images using virtual monochromatic
reconstructions. Challenges for the application of DECT
include temporal resolution of the data collection, image
noise, scattered radiation in wide volume scanning and
patient dose considerations. From an engineering
viewpoint these challenges translate to X-ray tube and
detector technology, as well as computer processing
speed and optimization. In radiology DECT has become
routine tool for certain imaging tasks where material
characterization and quantification is important for
diagnostics.
SP-0023 CT image quality: Using a model observer for
clinically relevant optimisation
N. Ryckx
1
, D. Racine
1
, A. Ba
1
, A. Viry
1
, F. Bochud
1
, F.R.
Verdun
1
1
CHUV - Institute of Radiation Physics IRA, Department of
Radiology, Lausanne, Switzerland
Introduction
The number of computed tomography (CT) examinations
has been increasing steadily in the last twenty years, and
this trend does not seem to slow down. For example, the
number of diagnostic CT examinations has increased by
17% between 2008 and 2013 in Switzerland. Furthermore,
the purpose of a CT has been extended to further
diagnostic and/or therapeutic procedures: Attenuation
correction for molecular imaging in nuclear medicine,
interventional CT fluoroscopy procedures and radiation
therapy treatment planning. Recently, the introduction of
iterative reconstruction algorithms allows for a potential
dose reduction by artificially removing image noise.
However, the risk of reducing radiation dose too low is to
potentially remove vital diagnostic information while
conserving the subjective visual aspect (especially in
terms of image noise) of images acquired at higher dose
but without iterative reconstruction. Finally, the usual
image quality metrics (CNR, MTF, NPS) are less pertinent
within the iterative reconstruction paradigm, because
these reconstructions are highly non-linear and non-
stationary.
Materials and methods
We seek to adapt image quality using clinically relevant
metrics. For this purpose, we use model observers, that
are in-silico image observers based on psychophysics and
statistical decision theory. The four cornerstones of a
model observer are the following:
-A clinically relevant task (lesion detection, lesion
localisation, etc.)
-An observer (human or in-silico)
-An adequate set of images, with a representative
statistical fluctuation
-A figure of merit (FOM) to quantify the observer
performance
In our approach, we evaluate CT image quality using a CHO
(channelized Hotelling observer) with dense difference of
Gaussian (DDoG) channels for low-contrast spherical lesion
detection in the abdominal region (e.g. focal liver lesions)
or a NPWE (non-pre-whitening matched filter with eye
filter) observer for high contrast lesion (e.g. renal stone)
detection. The images used for quality assessment are CT
axial slices performed on either an anatomical abdomen
phantom (QRM, Moehrendorf, Germany) with low-contrast
spherical targets of known size (5 and 8 mm diameter) and
contrast (-10 or -20 HU) or a home-made high-contrast
phantom, consisting of three cylinders (diam. 10 cm) of
different materials – Teflon for bone, polyethylene for fat
and PMMA for soft tissue – immersed in a cylindrical water
tank. The FOM used for quality assessment is either the
area under the ROC (receiver operating characteristic)
curve (AUC) or a detectability index d’. These assessments
were performed on 70 CT units in Switzerland.
Results and discussion
For abdominal low contrast targets, no significant
differences in AUC were noted between images
reconstructed by standard filtered back-projection (FBP)
and iterative reconstruction, but the dose-slice thickness
product (DSP, used as a normalised dose metric) varied
from 2.6 to 61 mGy mm, with reconstructed slice
thicknesses ranging from 2 to 5 mm. For the 5mm/20HU
target, 49% of the CT units gathered around an AUC range
of 0.86-0.98 for a DSP range of 5-20 mGy mm.
Nevertheless, 10% of the CTs were outliers because of
relatively high dose levels and limited AUC scores, for