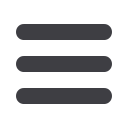

S256
ESTRO 36 2017
_______________________________________________________________________________________________
adjacent to or surrounding the bronchi. Fisher’s exact test
was used for comparison. p<0.05 was considered
significant.
Results
Fifty three patients (32%) were adapted due to changes in
A (8%), T (6%), N (15%) or T+N (3%), and 7% had more than
one replan. Atelectasis was seen at planningCT in 50
patients (30%) while in 12 patients (7%), it appeared during
RT. Presence of A before or during RT was not significantly
correlated with replanning. However, the changes in A
during RT significantly increased the probability of
replanning. (p=0.03), see Fig.2. Additionally, A within
5mm of T or N was significant (p=0.01). Only 11 patients
(6%) had changes in PE, but only in one patient was
replanning indicated. Patients with T0 or N0 had a
significant low risk of replanning (p=0.01, p=0.03) while
patients with two or more N had a high rate of replanning
(ns). Nodes in stations 1,2,3 or 4,7 or 10,11,12 had
significantly higher rate of replanning as compared to
patients with nodes in station 5,6 and 8,9. Node-volume
>30cm
3
had a significantly higher rate of replanning
(p=0.02). No correlation was found for T location, T size,
T or N adjacent to bronchi or for T or N shrinkage.
Histology was not significant for replanning. The imaging
rate may be decreased for patients with T0 and no A, as
none of these were adapted. For patients with N0 and no
A, only 11%, were replanned. On the contrary, 60% of the
patients with A and N volume>30cm
3
were replanned.
Conclusion
Prognostic factors for replanning of lung cancer patients
are changes in A during RT, A in the vicinity of T or N, large
N volume, and the N stations involved. No correlation
between risk of replanning and T size or T location was
found. The imaging frequency may be adjusted based on
these pre-treatment characteristic. Patients without A
and T0 or N0 had little risk of replanning. The imaging
frequency may be reduced for these patients, while
patients with A and large N volumes should be monitored
closely.
OC-0488 Thoracic tumor treatment course assessment
based on 4D dose accumulation for scanned proton
therapy
A. Meijers
1
, C. Richter
2
, F. Dessy
3
, J. Widder
1
, E.
Korevaar
1
, A. Jakobi
2
, C. Ribeiro
1
, J. Langendijk
1
, A.
Knopf
1
1
University of Groningen - University Medical Center
Groningen, Department of Radiation Oncology,
Groningen, The Netherlands
2
OncoRay
- National Center for Radiation Research in
Oncology, Faculty of Medicine and University Hospital
Carl Gustav Carus- Technische Universität Dresden-
Helmholtz-Zentrum Dresden
- Rossendorf, Dresden,
Germany
3
Ion Beam Applications IBA, Clinical Solutions, Louvain-
la-Neuve, Belgium
Purpose or Objective
With the increase of proton therapy facilities worldwide
featuring Pencil Beam Scanning (PBS) as their only
treatment modality, PBS is on the way of becoming the
standard for proton therapy. However, for some
indications in the thoracic region PBS is not widely used
due to uncertainties in the planned dose, which can be
caused by combined effects of setup errors, range
uncertainty, interplay effect, breathing irregularity,
anatomical variations, delivery machine uncertainties,
etc. By performing pre-treatment plan robustness
evaluation that includes these effects, it is evident that
actual delivered fractional dose at any instance is highly
uncertain to predict. 4D dose accumulation is able to
control some of the uncertainties that are affecting pre-
treatment evaluation of the plan quality. Therefore, the
purpose of this proof-of-concept study is to investigate the
feasibility of monitoring and assessing the quality of
delivered treatment fractions throughout the treatment
course.
Material and Methods
4D dose accumulation is performed by utilizing (1) delivery
machine log files (IBA, Belgium), (2) breathing pattern
records (ANZAI, Japan) and (3) planning 4DCT scans or
repeated 4D control CT scans (Siemens, Germany). Dose
computation is performed in the RayStation (RaySearch,
Sweden) treatment planning system (TPS).
For every spot that is delivered during a particular
fraction, the spot energy, position, dose (as charge) and
absolute time of delivery is retrieved from the machine
log file using a dedicated script. Patient’s breathing
pattern is analyzed and inhale peaks are determined.
Subsequently, all breathing cycles are divided in 10 phases
and each phase is associated with absolute time. PBS spots
are split in 10 groups according to their corresponding
phase and written to 10 treatment sub-plans (DICOM),
where every sub-plan corresponds to a particular phase of
the 4DCT. Using scripting capabilities of the TPS, sub-plans
are imported for dose computation. Eventually dose
warping to the reference phase is performed to estimate
the delivered fractional dose.
Data sets used for the proof-of-concept were not collected
during the same treatment fraction.
Results
By using the described method the timeline of a PBS
delivery can be correlated with patient’s breathing
pattern as shown in Figure 1. Computation of log based
sub-plans on 4DCT results in an accumulated fractional 4D
dose distribution as shown in Figure 2. Based on the
exemplary case, the method allows to assess the
conformity between planned and delivered doses (i.e.,
CTV V95 has dropped to 96.7% from nominal 100%).