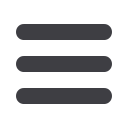

S295
ESTRO 36 2017
_______________________________________________________________________________________________
The first part of the lecture will focus on challenges and
recent
recommendations
for
isolation
and
characterisation of exosomes and their cargo in preclinical
models and human biofluids, while the second part will
present the latest developments within exosome research
relevant to radiation oncology.
1. Andaloussi, et al. Nature Rev Drug Discov 2013;12:347–
357.
2. Muralidharan-Chari et al. J Cell Sci 2010;123:1603–
1611.
3. Mitchell et al. Proc Natl Acad Sci 2008;105:10513–
10518.
4. King KW et al. BMC Cancer 2012;12:421.
5. Park et al. Mol Cell Proteomics 2010;9:1085–1099.
6. Li et al. Cancer Res 2016;76(7):1770–1780.
7. Kucharzewska et al. Proc Natl Acad Sci
2013;110(18):7312–7317.
Teaching Lecture: Update on molecular radiotherapy
SP-0558 Update on molecular radiotherapy
G. Flux
1
1
The Institute of Cancer Research and The Royal Marsden
NHS Foundation Trust, Department of Nuclear Medicine,
Sutton, United Kingdom
Molecular radiotherapy was first developed following a
meeting 80 years ago between the clinician Saul Hertz and
the physicist Karl Compton after a lunchtime symposium
on ‘What Physics can do for Biology and Medicine’.
Radioiodine was synthesised and used to study thyroid
metabolism and to treat both benign and malignant
thyroid disease. In the first treatments, full dosimetry was
performed by the physicist Arthur Roberts, using the best
technology available at the time, 10 years before the
development of the Anger camera. The use of
radiotherapeutics for oncology continues to expand. In
particular Ra-223, used to treat bone metastases from
castration resistant prostate cancer, has now been
approved in some countries. In the UK alone, treatments
doubled in the year before approval. Administrations
continue to be performed without imaging or patient
specific dosimetry, although there are an increasing
number of studies to show that this is feasible and that
there exists a correlation between the absorbed doses
delivered and response. A recent retrospective study of
Re-186 HEDP demonstrated that survival was improved
with the administration of higher activities and that the
absorbed doses delivered to whole-body and to tumours
correlated inversely with survival. This is due to the
uptake being a marker of tumour burden, indicating the
challenging potential of theragnostics. Lu-177 PSMA is
being used increasingly for the treatment of bone
metastases. Initial trials have shown safety and efficacy,
and it is likely that its use will increase dramatically in the
coming years. This follows results from the NETTER study
where Lutathera was found to significantly improve PFS
when compared with Sandostatin LAR (Octreotide LAR) in
patients with advanced midgut neuroendocrine tumors
(NETs). The treatment of liver metastases with Y-90
microspheres continues to expand. Internal dosimetry is
playing a larger role for both glass and resin microspheres
and a dosimetry software package has been developed by
BTG for Theraspheres. Following many years of ad hoc
treatment regimens, without specific international
guidelines, a number of dosimetry-based clinical trials are
now in progress or development, focussed on radioiodine
treatment for thyroid cancer, Lu-177 DOTATATE for adult
and paediatric neuroendocrine tumours, and I-131 mIBG
for neuroblastoma. The European basic safety standards,
mandating dosimetry based treatments and verification
for molecular radiotherapy as well as for external beam
radiotherapy, are due to be enacted in February 2018. The
EANM has a task force specifically addressing this issue and
have recently conducted the first European survey of MRT.
This has found a wide discrepancy in practice. As the
treatment of cancer with radiotherapeutics is
acknowledged to be a form of radiotherapy, many
scientific, logistical and political challenges must be
addressed. This field epitomises the need for a
multidisciplinary approach to improve clinical practice for
the benefit of the patient.
Teaching Lecture: Basics, implementations,
applications and limitations of Monte Carlo dose
calculation algorithms
SP-0559 Basics, implementations, applications and
limitations of Monte Carlo dose calculation algorithms
F. Verhaegen
1
1
Maastricht Radiation Oncology MAASTRO GROW - School
for Oncology and Developmental Biology- University
Maastricht, Maastricht, The Netherlands
Monte Carlo (MC) simulations are potentially the most
accurate and powerful techniques to calculate dose in
radiotherapy, in addition to other quantities of interest,
e.g. particle fluence. They are increasingly being used in
treatment planning systems (TPS) for photon, electron and
proton therapy. In addition, they are also increasingly
encountered in preclinical research that involves precision
irradiation of small animals, which nowadays use
dedicated MC TPS available for radiobiology research.
This lecture will cover the basics of MC particle transport:
transport mechanisms, sampling from cross sections and
definition of complex geometries. The advantage of track
visualization and particle tagging will be demonstrated.
The broad applications of MC will be discussed in
modelling radiation detectors, modelling radiation sources
(linear accelerators, proton beam lines, brachytherapy
sources), dose calculation in cancer patients, and dose
calculation in imaging panels for radiotherapy. In dose
calculation in cancer patients, the subject of dose
reporting as dose to water or dose to medium will be
mentioned. MC simulations are also used to predict e.g.
the emission of prompt gamma photons or annihilation
photons in proton beams, to verify the proton range,
which requires knowledge of nuclear interactions.
Besides radiation dose, MC simulations can easily yield
particle fluence spectra, which may be used for dose
conversions, or as input for calculations of biological
damage and relative biological effectiveness. Other
specialized applications involve using MC for modelling
precision irradiators and planning the irradiation of small
animals for preclinical research. The accurate dose
calculations of kV photon beams are needed to mimic
human radiotherapy at the scale of small animals. Also the
complex interactions of secondary electrons released in
photon beams with the magnetic field inside an MR-linac,
requires MC modelling for accuracy. MC dose calculations
also lend themselves to modelling dynamic geometries.
Limitations of MC methods are that they are still relatively
slow, they exhibit statistical noise and they may express
dose in unfamiliar units (dose to medium instead of dose
to water).
Teaching Lecture: RTTs roles and responsibilities to
support future practice
SP-0560 RTTs roles and responsibilities to support
future practice
M. Coffey
1
1
Coffey Mary, Dublin, Ireland