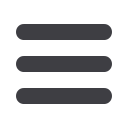

S475
ESTRO 36 2017
_______________________________________________________________________________________________
protons, IMPT
PR
plans were the most robust and only 4/26
(15%) decreased in coverage to below 99%. The CTV
coverage for all patients and plans are shown in Fig 1. The
most common anatomical changes are lateral target
deformations, enlargement of mediastinum and changes
in diaphragm position. The posterior proton plans are
sensitive to target deformations, while the multiple
photon fields are sensitive to all three types of changes
(see
Fig.
2).
Conclusion
Treating oesophageal cancer with protons has the
advantage of decreasing dose to organs at risk and at the
same time it improves the robustness towards common
anatomical changes. Frequent imaging is still needed to
identify patients with target deformations requiring
adaptive treatment planning.
PO-0878 Plan adaptation on the MR-Linac: first
dosimetric validation of a simple dose shift
R. Koopman
1
, A.J.A.J. Van de Schoot
1
, J. Kaas
1
, T. Perik
1
,
T.M. Janssen
1
, U.A. Van der Heide
1
, J.J. Sonke
1
1
Netherlands Cancer Institute Antoni van Leeuwenhoek
Hospital, Radiation Oncology, Amsterdam, The
Netherlands
Purpose or Objective
Patient positioning on the MR-Linac (MRL; Elekta AB,
Stockholm) requires online plan adaptations to correct for
setup errors due to the fixed couch position. The aim of
our study was to validate the size and direction of such
plan adaptations (simple dose shifts) and evaluate
dosimetric differences for rectum cancer patients.
Material and Methods
The planning CT and delineated structures of four rectum
cancer patients were selected. For each patient, a MRL
treatment plan was generated with Monaco using a 7-
beam IMRT technique (25 x 2.0 Gy) including all MRL-
specific properties (7MV, 1.5 T magnetic field, collimator
90°, FFF, SAD: 143.5 cm). Patient setup errors of 1.0 cm
and 2.0 cm in the CC and LR directions were simulated by
shifting the planning CT with respect to the isocenter
position. For each setup error, the initial plan was adapted
by first adjusting the leaves of each segment to
approximate the shift and second re-optimize the weight
of each segment. Also, a reference plan was generated by
adapting the initial plan with a 0.0 cm shift, as the second
phase of plan adaptation was observed to introduce dose
changes even for a 0.0 cm shift. All plans were rescaled
(PTV V
95%
= 99%). The reference and adapted plans were
irradiated on the MRL on a slab phantom with a 2D
detector array (PTW Octavius 1500
MR
) inserted parallel to
the couch at the center position of the PTV. For each plan,
the phantom position was changed according to the
introduced shift. Patient setup errors in the AP direction
cannot be evaluated using this measurement setup. The
measured 2D dose distribution of the reference plan was
rigidly registered to the measured 2D dose distribution of
the adapted plans in order to assess the positional
accuracy of the simple dose shift. After alignment, the
similarity between the 2D dose distributions of the
reference plan and the adapted plans was evaluated using
a 3%/3mm γ analysis (local dose, 20% low dose threshold).
Results
For all adapted plans, the measured positional accuracy
was within 0.1 cm. The γ analysis between the dose
distributions of the reference plan and the adapted plans
resulted in an average pass rate (γ≤1) of
96.2% (range: 83.3% – 99.9%). Smaller values of γ
mean
were
observed for dose shifts in the CC direction compared to
the LR direction as well as for 1.0 cm dose shifts compared
to 2.0 cm dose shifts (Table 1). Figure 1 shows an example
of a 2D γ distribution. High γ values are measured in the
low dose area mainly. A simple dose shift to correct for a
1.0 cm setup error in the CC direction resulted in limited
dose differences. Various γ hotspots were observed for
the 2.0 cm setup error in the LR direction.