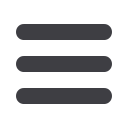

S479
ESTRO 36 2017
_______________________________________________________________________________________________
Conclusion
Our novel binnin g strategy for 4DMRI outperformed the
classical strategies, resulting in a 4DMRI with h igh
precision and fewer artefacts in the presence of irregular
breathing.
PO-0882 Proxy-free slow-pitch helical 4DCT
reconstruction
R. Werner
1
, C. Hofmann
2
, T. Gauer
3
1
University Medical Center Hamburg-Eppendorf,
Department of Computational Neuroscience, Hamburg,
Germany
2
Siemens Healthcare, Imaging & Therapy Systems,
Forchheim, Germany
3
University Medical Center Hamburg-Eppendorf,
Department of Radiotherapy and Radio-Oncology,
Hamburg, Germany
Purpose or Objective
Standard 4DCT protocols correlate external breathing
signals (exploiting e.g. surface tracking devices or
abdominal belts) to raw or reconstructed image data to
allow for reconstruction of a series of CT volumes at
different breathing phases. From a radiotherapy (RT)
workflow perspective, dealing with external devices for
breathing signal recording is cumbersome. Moreover, if
the respiratory signal is corrupted, 4DCT reconstruction is
not possible at all. At this, proxy-free reconstruction – i.e.
4DCT reconstruction without using an external breathing
signal – could improve RT workflows. We present a novel
approach for slow-pitch helical 4DCT reconstruction and
illustrate its feasibility.
Material and Methods
Similar to standard external breathing signal-driven slow-
pitch helical CT we assume a sufficiently low pitch and
gantry rotation time to be given to ensure existence of
appropriate raw data for reconstruction of image data at
each z position and desired breathing phase. We then
pursue a three-step process: (1) image-based derivation of
a differential breathing signal; (2) correlation of the
extracted breathing signal to raw data; and (3) integration
and minimization of an artifact-metric into the final (here:
phase-based) reconstruction process. For the crucial step
(1), we initially reconstruct slices at a series of z-positions
and points in time and determine (slice wise, averaged
over a specific region of interest) the change Δ
torso
/Δt in
chest wall height. As Δ
torso
/Δt can be considered as
derivation of the desired breathing signal (figure 1); its
zero-crossings represent the end-inspiration (and the end-
expiration) breathing phases to be correlated to the raw
data.
Feasibility of the afore-mentioned approach is
investigated using routinely acquired 4DCT lung and liver
data sets. A detailed analysis of motion dynamics and
image artifacts is performed in proxy-free reconstructed
4DCT data sets of three patients and resulting numbers are
compared to corresponding standard external breathing
curve-driven phase-based (PB) reconstructions based on
the same 4DCT raw data plus RPM breathing signal.
Results
Figure 2 illustrates that proxy-free and common external
breathing signal-driven PB-reconstructed 4DCT data are
comparable both in terms of image quality and
represented motion amount. In detail, the considered
proxy-free datasets contained approximately 5% more
artifacts than the PB data sets. Differences of represented
tumor mass center motion as well as the amount of e.g.
diaphragm motion between end-inhalation and -
exhalation were negligible (max. 1 voxel).
Conclusion
We presented a novel approach for proxy-free slow-pitch
helical 4DCT reconstruction and illustrated its feasibility.
Although the proxy-free reconstructed images contain
slightly more motion artifacts, we consider the approach
to be helpful especially in the case of corrupted breathing
signals recordings (no need for re-scanning the patient).
PO-0883 Clinical Implementation Model-Based CT to
Replace 4DCT for Lung Cancer Treatment Planning
D. Low
1
, D. O'Connell
1
, L. Yang
1
, J. Lewis
1
, P. Lee
1
1
UCLA Medical Center, Department of Medical Physics, Los
Angeles, USA
Purpose or Objective
To implement motion-model based CT into clinical
practice, replacing 4DCT for breathing motion
management treatment planning.
Material and Methods
A breathing motion model that employs a mathematical
motion equation, two real-time breathing surrogates,
breathing amplitude and breathing rate, and employing
multiple fast helical, low-dose CT scanning has been
introduced into clinical practice. The imaging process uses
a bellows-based system to monitor the breathin g cycle,
which is defined as the amplitude and rate of the bellows
signal. The fast helical CT scans are reg istered to
determine the lung tissue positions, correlate d to the
breathing amplitude and rate on a slice-by-s lice basis. A
published motion equation is employed to characterize
the motion for each voxel. The motion model is employed
to reconstruct the original fast helical CT scans and the
original and reconstructed scans compared to determine
the overall model motion prediction accuracy. Eight
amplitude-based CT images are constructed and sent to