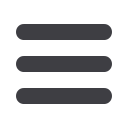

S901
ESTRO 36 2017
_______________________________________________________________________________________________
performed. The maximum (D
2%
), minimum (D
98%
) and
median (D
50%
) doses were registered.
Results
The maximum HU average difference over all the patients
was observed in the thyroid (81.37 ± 36.01 HU) followed
by the PTV50 (10.76 ± 15.70 HU) and the parotids (9.39
±16.01 HU). The differences found with the AAA®
algorithm were below 0.1% for D
2%
, D
98%
and D
50%
in target
volumes and between -0.11 and 0.36% in OAR. The
differences observed with Acuros XB® Algorithm were less
than 0.2% in target volumes and 0.31% in OAR. Moreover,
the differences between two algorithms were statistically
insignificant (p > 0.4).
Conclusion
This study shows that the use of intravenous contrast
during CT simulation does not significantly affect dose
calculation in head and neck VMAT plans using AAA and
Acuros XB algorithms.
EP-1676 Comparison of accuracy of Hounsfield units
obtained from pseudo-CT and true CT images
N. Reynaert
1
, P.F. Cleri
1
, J. Laffarguette
1
, B. Demol
1
, C.
Boydev
1
, F. Crop
1
1
Centre Oscar Lambret, PHYSIQUE MEDICALE, Lille,
France
Purpose or Objective
Quality of pseudo-CT (pCT) images used for MRI-only
treatment planning is often evaluated using the so-called
MAE (Mean Average Energy) curve. Furthermore, a
dosimetrical comparison is performed by comparing DVHs
using pCT and true CT (tCT). The tCT is always considered
as the reference, while uncertainties on these images are
neglected. The purpose of the current work is to compare
MAE curves for tCT images by varying different scanning
parameters and to compare the results with uncertainties
on our pCTs.
Material and Methods
A Toshiba Large Bore CT was used. Different IVDT curves
were determined, for different energies (100-135 kV),
FOVs, reconstruction kernel, phantom size, insert
positions, using an in-house phantom, with variable size.
The IVDT curves were used in our in-house Monte Carlo
platform for recalculation of Cyberknife and Tomotherapy
plans. pCT images were generated from MRI images (3D T1
sequence) using an atlas-based method. Image quality was
determined using MAE, ME and gamma curves.
Results
Three parameters for tCT had an important impact on the
HUs, namely the energy, patient size and reconstruction
kernel. These parameters individually modified image
values with up to 300 HUs in bone inserts. Furthermore,
patient size and energy are often correlated as, it is
specifically for small patients that lower energies are
used, both leading to higher HUs in bone. The impact of
the reconstruction kernel was a surprise (e.g. comparing
the FC64 and FC13). For the energy and the reconstruction
kernel one can consider introducing specific IVDTs. It
becomes more complicated when the IVDT should be
modified as a function of patient diameter though.
Furthermore, in some TPSs (e.g. Masterplan, Nucletron)
only one predefined IVDT is used. Another important
problem is the fact that the HUs in the air surrounding the
patient are increased when using large phantom sizes
(changing from -1000 HU to -910 HU). Depending on the
IVDT, this can lead to a largely overestimated air density
around the patient (0.2 g/cm
3
) with a possible dosimetric
impact. The dosimetric impact of using different IVDTs
when modifying energy, reconstruction kernel and patient
size individually are below 2 %, for all Cyberknife and
Tomotherapy plans considered. This is also the case for
most of our pCT images. In extreme cases for tCT, e.g.
when comparing a small patient scanned at 100 kV using
the FC64 reconstruction kernel compared to a large
patient scanned at 135 kV using the FC13 kernel, HU
differences up to 900 (in bone) can be obtained leading to
systematic dose differences up to 6 % (DVH shift). Using an
“average” IVDT still leads to dose uncertainties > 2 %.
Results can be CT scanner specific.
Conclusion
Uncertainties on pCT images used for MRI-only treatment
planning should be compared to those on tCT images. The
uncertainties on tCT images (even when not considering
CT artifacts) are non-negligible and are of the same order
as those on pCT images generated by e.g. atlas-based
methods.
Electronic Poster: Physics track: (Quantitative)
functional and biological imaging
EP-1677 Multicentre initiative for standardisation of
image biomarkers
A. Zwanenburg
1
, Image Biomarker Standardisation
Initiative IBSI
2
1
OncoRay - National Center for Radiation Research in
Oncology, Faculty of Medicine and University Hospital
Carl Gustav Carus - Technische Universität Dresden -
Helmholtz-Zentrum Dresden-Rossendorf, Dresden,
Germany
Purpose or Objective
Personalised cancer treatment has the potential to
improve patient treatment outcomes. One particular
approach to personalised treatment is radiomics.
Radiomics is the high-throughput analysis of medical
images. There are several challenges within the radiomics
field which need to be overcome to translate findings into
clinical practice. The Image Biomarker Standardisation
Initiative (IBSI) addresses the challenge of reproducing and
validating reported findings by comparing and
standardising definitions and implementation of several
image feature sets between participating institutions.
Material and Methods
A 5x4x4 voxel digital phantom was devised, with a super-
imposed region-of-interest (ROI) mask (Figure 1). This
volume has characteristics similar to real patient volumes
of interest, namely voxels outside of the ROI and missing
grey levels. The phantom is moreover sufficiently small to
manually calculate features for validation purposes.
Because no pre-processing steps (e.g. discretisation) are
necessary for calculations on the phantom, feature values
may be standardised across all institutions.