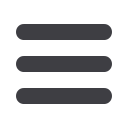

S85
ESTRO 36 2017
_______________________________________________________________________________________________
higher values between 2-10
keV.µm-1
can be found in
treatment plans.
Methods and Results
:
Studies concluding that the mid-SOBP relative biological
effect (RBE) of protons is 1.1 for all tissues and tumours
at all doses per fraction have recently been criticised due
to their use
of:
1. kilovoltage x-ray controls (which mostly provide RBE
values less than 1 and should be excluded),
2. a very limited number of cell lines,
3. a predominance of high doses per fraction (as used for
eye melanomas),
4. linear-only fitting (rather than linear quadratic),
5. animal based studies that used only acute reacting
tissues (with high α/β ratios), known to show little RBE
change with dose per fraction when using fast neutrons
(which ionise mostly by forming recoil protons).
No classical late reacting (low α/β) tissue RBEs have been
published so far: it is these tissues that will influence PT
late effects for important normal tissues within the PTV
and closely around it. Of prime concern is neurological
tissue with α/β of 2 Gy. Using a scaling model based on
the original work of Wilkens & Oelfke, but with added
saturation effects for increases in both α and β with LET,
figures 1 and 2 shows the predicted RBEs in the range of
LET normally in the SOBP
(1-2keV.µm-1
) and the general
increase in RBE with LET and decrease of RBE with dose
per fraction; at higher values of LET (2-10) further
increases in RBE occur, in some cases to beyond 2 at LETs
of 6-10.
Outside the brain, other normal tissue types may carry
lesser importance so that, for example, a slightly raised
RBE in muscle may not produce enhanced late effects in a
very confined volume, as may serially organised tissues
such as lung and liver, but cardiac tissue, bowel and
kidney remain at risk depending on the volume irradiated.
One intriguing aspect is the fall of RBE with increased dose
per fraction, especially in tisses with low α/β values,
which may encourage the use of carefully estimated
hypofractionated total doses, using BED equations with
imbedded RBE limits: the RBEmax and RBEmin
(respectively reflecting the change in α and β with LET):
Figures 1 and 2 show how different α/β ratio bio-systems
may behave with the lowest α/β system crossing over to
have the lowest RBE at higher doses. Values lower than
1.1 can occur in high α/β systems, with risk of
underdosage if a 1.1 RBE is used.
Conclusions
. There should be no complacency about RBE
values, even within SOBP`s: 1.1 is not be appropriate.
These higher values may explain some reported adverse
toxicities following PT, such as necrosis of the optic
chiasm and temporal lobe, and failure to cure some very
radiosensitive tumour types with high α/β (lymphomas and
many childhood cancers). Comprehensive RBE studies are
urgently indicated.
References:
Jones, B in Cancers (Basle) 2015, 7, 460-480;
also, Brit J Radiol, Why RBE must be a variable and not a
constant. Published Online: May 05, 2016.
Figures 1&2
SP-0169 A small animal tumour model for low-energy
laser-accelerated particles
J. Pawelke
1,2
, K. Brüchner
3,4
, M. Krause
4,5,6
, E. Leßmann
2
,
M. Schmidt
5
, E. Beyreuther
2
1
OncoRay - National Center for Radiation Research in
Oncology- Faculty of Medicine and University Hospital
Carl Gustav Carus-Technische Universität Dresden,
Department of Medical Physics- Laser Radiooncology
Group, Dresden, Germany
2
Helmholtz-Zentrum Dresden - Rossendorf, Institute of
Radiation Physics, Dresden, Germany
3
Faculty of Medicine and University Hospital Carl Gustav
Carus- Technische Universität Dresden, Experimental
Center, Dresden, Germany
4
Helmholtz-Zentrum Dresden - Rossendorf, Institute of
Radiooncology, Dresden, Germany
5
OncoRay - National Center for Radiation Research in
Oncology- Faculty of Medicine and University Hospital
Carl Gustav Carus-Technische Universität Dresden,
Department of Radiation Oncology, Dresden, Germany
6
German Consortium for Translational Cancer Research
DKTK and German Cancer Research Center DKFZ,
Dresden Site, Dresden, Germany
Introduction:
The long-term aim of decveloping laser-
based acceleration of protons and heavier ions towards
clinical radiation therapy application requires not only
substantial technological progress, but also the
radiobiological characterization of the resulting ultra-
short and ultra-intensive particle beam pulses. Recent in
vitro data showed similar effects of laser-accelerated
versus conventional proton beams on clonogenic cell
survival and DNA double-strand breaks. As the proton
energies currently achieved for radiobiological
experiments by laser-driven acceleration are too low to
penetrate standard tumour models on mouse legs, a small
animal tumour model allowing for the penetration of low
energy protons (~20 MeV) was developed to further verify
the effects in vivo.
Methods:
The mouse ear tumour model was established
for human HNSCC FaDu and human glioblastoma LN229
cells. For this, cells were injected subcutaneously in the
right ear of NMRI nude mice and the growing tumours were
characterized with respect to growth parameters and
histology. After optimizing the number of injected cells
and used medium (PBS, Matrigel) the radiation response
was studied by 200 kV X-ray irradiation. Furthermore, a
proof-of-principle full scale experiment with laser-
accelerated electrons was performed to validate the FaDu