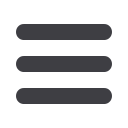

S912
ESTRO 36
_______________________________________________________________________________________________
EP-1673 Electron-density assessment using dual-energy
CT: accuracy and robustness
C. Möhler
1,2
, P. Wohlfahrt
3,4
, C. Richter
3,4,5,6
, S. Greilich
1,2
1
German Cancer Research Center DKFZ, Division of
Medical Physics in Radiation Oncology, Heidelberg,
Germany
2
National Center for Radiation Research in Oncology
NCRO, Heidelberg Institute for Radiation Oncology HIRO,
Heidelberg, Germany
3
OncoRay - National Center for Radiation Research in
Oncology, Faculty of Medicine and University Hospital
Carl Gustav Carus- Technische Universität Dresden-
Helmholtz-Zentrum Dresden - Rossendorf, Dresden,
Germany
4
Helmholtz-Zentrum Dresden-Rossendorf, Institute of
Radiooncology, Dresden, Germany
5
Department of Radiation Research in Oncology, Faculty
of Medicine and University Hospital Carl Gustav Carus-
Technische Universität Dresden, Dresden, Germany
6
German Cancer Consortium DKTK, Dresden, Germany
Purpose or Objective
Current treatment planning for essentially every external
radiation therapy (photons, electrons, protons, heavier
ions) is not able to account for patient-specific tissue
variability or non-tissue materials (e.g. implants, contrast
agent) which can lead to considerable differences in dose
distributions (figure 1). This is due to the conversion of CT
numbers to electron density or stopping power using a
heuristic Hounsfield look-up table. In contrast, dual-
energy CT (DECT) allows for a patient-specific
determination of electron density – the only (most
important) parameter influencing photon (ion) dose
distributions. Among the many algorithms proposed for
this purpose, a trend towards increased complexity is
observed, which is not necessarily accompanied by
increased accuracy and might at the same time militate
against clinical implementation. Here, we therefore
investigated the performance of a seemingly simple
linear-superposition method (Saito, 2012, Hünemohr et
al.,
2014).
Material and Methods
Key feature of the studied approach is a parameterization
of the electron density, given by 'alpha blending” of the
two DECT images. The blending parameter can be
obtained by empirical calibration using a set of bone tissue
surrogates and a linear relationship between relative
photon absorption cross sections of the higher and lower
voltage spectrum. First, this linear relation was analyzed
to quantify the purely methodological uncertainty (i.e.
with ideal CT numbers as input), based on calculated
spectral-weighted cross sections from the NIST XCOM
database for tabulated reference tissues (Woodard and
White, 1986). A clear separation from CT-related sources
of uncertainty (e.g. noise, beam hardening) is hereby
crucial for a conclusive assessment of accuracy. Secondly,
we tested the proposed calibration method on published
DECT measurements of typical tissue-surrogate phantoms
and evaluated its uncertainty.
Results
The methodological uncertainty of electron-density
assessment for the alpha-blending method was found to
be below 0.15% for arbitrary mixtures of human tissue. In
the case of small abundance of high-Z elements, electron-
density results are positively biased, e.g. 0.5% for thyroid
containing 0.1% iodine (Z=53) by mass, which is due to the
K edge of the photoelectric effect. The calibration
parameters obtained from various published data sets,
showed very little variation in spite of diverse
experimental setups and CT protocols used. The
calibration uncertainty was found to be negligible for soft
tissue while it was dominated by beam hardening effects
for bony tissue.
Conclusion
The alpha-blending approach for electron-density
determination shows universal applicability to any mixture
of human tissue with a very small methodological
uncertainty (< 0.15%); and a robust and bias-free
calibration method, which is straightforward to
implement. We conclude that further refinement of
algorithms for DECT-based electron-density assessment is
not advisable.
EP-1674 Experimental investigation of CT imaging
approaches to deal with metal artefacts in proton
therapy
S. Belloni
1,2
, M. Peroni
1
, S. Safai
1
, G. Fattori
1
, R. Perrin
1
,
M. Walser
1
, T. Niemann
3
, R.A. Kubik-Huch
3
, A.J. Lomax
1
,
D.C. Weber
1,4,5
, A. Bolsi
1
1
Paul Scherrer Institut, Center for Proton Therapy,
Villigen PSI, Switzerland
2
University of Bologna, Department of Physics and
Astronomy, Bologna, Italy
3
Cantonal Hospital Baden, Department of Radiology,
Baden, Switzerland
4
Inselspital, Radiation Oncology, Bern, Switzerland
5
University Hospital Zurich, Radiation Oncology, Zurich,
Switzerland
Purpose or Objective
Metal implants are challenging for proton therapy, mainly
because of beam hardening artefacts severely
compromising image quality of the planning CT. In fact,
they result in non-negligible uncertainties in Stopping
Power (SP) evaluation and significantly affect VOI
delineation accuracy. The aim of this study was to
compare different approaches to minimize the artefacts:
a manual approach based on delineation of the visible
artefacts, which was developed and is used clinically at
the Center for Proton Therapy (PSI), and the new tools
recently introduced in CT, such as SIEMENS Iterative Metal
Artefact Reduction (iMAR) and Sinogram Affirmed Iterative
Reconstruction (SAFIRE). Moreover, an experimental
verification of direct SP calculation from Dual Energy (DE)
images with iMAR has also been considered.
Material and Methods
A clinical treatment of a cervical chordoma patient was
reproduced on a head and neck anthropomorphic
phantom, which presents metal implants (titanium screws
and cage) in the area where the PTV was defined. An IMPT
plan with two anterior oblique and two posterior oblique
fields (dose per fraction 2 GyRBE) was optimized and
calculated on 7 different CTs which corresponded to the
different imaging approaches: no correction of artefacts,
manual correction, iMAR (each of these reconstructed
using Filtered Back Projection (FBP) and SAFIRE) and DE