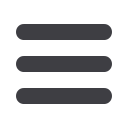

S177
ESTRO 36 2017
_______________________________________________________________________________________________
planned to confirm and consolidate correction factors and
determine the overall uncertainty on absorbed dose-to-
water obtained using each system. The next experimental
step is to perform the same experimental comparison for
a real clinical situation: a dose cube of 10 x 10 x 10 cm³,
created by a superposition of mono-energetic layers.
OC-0340 Validation of HU to mass density conversion
curve: Proton range measurements in animal tissues
J. Góra
1
, G. Kragl
1
, S. Vatnitsky
1
, T. Böhlen
1
, M.
Teichmeister
1
, M. Stock
1
1
EBG MedAustron GmbH, Medical Physics, Wiener
Neustadt, Austria
Purpose or Objective
Proton dose calculation in the treatment planning system
(TPS) is based on HU information taken from the CT scans
and its relation to the relative stopping powers (RSP).
However, tissue equivalent substitutes commonly used in
the process of conversion curve definition may not reflect
precisely the properties of real, human tissues. Therefore,
various animal tissues were used for validation of the CT
number to mass density (MD) conversion curves
implemented in the TPS (RayStation v5.0.2).
Material and Methods
10 animal tissue samples (pig) were used in this study
(muscle, brain, bone, blood, liver, spleen, lung, fat,
kidney and heart). Each sample was prepared and
wrapped separately. 3-4 tissues were placed in dedicated
phantoms (head and pelvis) at a time and CT scans were
taken in the clinically accepted planning protocols.
Specially designed PMMA phantoms where composed of
two parts: a) an internal box, which could fit the animal
tissues inside, b) the outer PMMA cover, designed to
simulate pelvis (see fig.1c) and head during CT scan. The
design of the phantoms not only helped to reduce imaging
artefacts but also allowed to apply a slight pressure on the
tissues in order to remove unwanted air. Subsequently,
the tissue phantom was attached to the front of the water
phantom, where with the use of 2 Bragg peak chambers,
range measurements were performed. All measurements
were performed within 24h after the animal was
slaughtered with the use of one, central, 160.3 MeV pencil
beam. For each sample, multiple irradiation positions
were chosen in a very precise matter, as it was extremely
important to choose the most homogeneous path through
which the proton beam would pass. Acquired CT data was
used to read out the HU, correlate them with the
measured RSP and validate against implemented CT
number to MD conversion curves.
Results
Figure 1, shows the comparison between measured RSP
and HU for real tissue samples and implemented
conversion curve in the TPS a), CT scan of the adult,
abdomen protocol b), and measurement set-up c). The
measured data for all soft tissues were found to be within
1% agreement with the calculated data. Only for lung
tissue the deviations were up to 3.5%. For bone, both the
difficulty in assessing the actual thickness of the part
where the beam was passing through, as well as the
inhomogeneous nature of this tissue, prevented us from
the accurate RSP assessment. However, for 2
measurements out of 3, the measured RSP where within
3.5% uncertainty.
Conclusion
The experimental validation of the conversion curve
resulted in good agreement between measured and
calculated data, therefore we can use it in the clinical set-
up with confidence. There is a number of uncertainty
sources related to these measurements, starting from HU
to RSP model, real tissue heterogeneities or uncertainties
related to acquisition of the CT data due to beam
hardening. The last one, we tried to minimize by using
especially dedicated phantom.
OC-0341 Monte Carlo dose calculations using different
dual energy CT scanners for proton range verification
I.P. Almeida
1
1
Maastricht Radiation Oncology MAASTRO clinic, Physics
Research, Maastricht, The Netherlands
Purpose or Objective
To simulate the dose profile for proton range verification
by means of Monte Carlo calculations and to quantify the
difference in dose using extracted values of relative
electron densities (
ρ
e
) and effective atomic numbers (
Z
eff
)
for three commercial dual-energy computed tomography
(DECT) scanners from the same vendor: a novel single-
source split-filter (i.e. twin-beam), a novel single-source
dual-spiral and a dual source device. This study aims also
to provide a comparison between the use of different
DECT modalities and the conventional single-energy CT
(SECT) technique in terms of dose distributions and proton
range.
Material and Methods
Measurements were made with three third generation
DECT scanners: a novel dual spiral at 80/140 kVp, a novel
twin-beam at 120 kVp with gold and tin filters, and a dual-
source scanner at 90/150kVp with tin filtration in the high
energy tube. Images were acquired with equivalent CTDI
vol
of approximately 20 mGy and reconstructed with
equivalent iterative reconstruction algorithms. Two
phantoms with tissue mimicking inserts were used for
calibration and validation. Monte Carlo proton dose
calculations were performed with GEANT4, in which the
materials and densities were assigned using the DECT
extracted values of
ρ
e
and
Z
eff
for both phantoms.
Simulations were done with monoenergetic proton beams
impinging under directions to the cylindrical phantoms,
covering different tissue-equivalent inserts. Dose
calculations were also performed on images from a third
generation SECT scanner at 120 kVp. Simulations based on
DECT and SECT images were compared to a reference
phantom.
Results
Range shifts on the 80% distal dose fall-off (R80) were
quantified and compared for the different beam directions
and media involved to a reference phantom. Maximum R80
range shifts from the reference values for the calibration
phantoms based on DECT images were 3.5 mm for the
twin-beam, 2.1 mm for the dual-spiral and for the dual-
source. For the same phantom, simulations based on SECT
images had a maximum range shift of 4.9 mm. 2D stopping
power maps were computed and compared for the
different techniques.