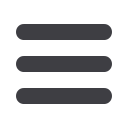

S179
ESTRO 36 2017
_______________________________________________________________________________________________
MC simulations accurately modelled the dose distribution
around the Bragg peak and can be used to estimate the
LET at any given position of the proton beam with
optimized parameters. The LET spectrum varied
considerably with depth and such LET estimates are highly
valuable for future studies of relative biological
effectiveness of protons.
OC-0343 Experimental setup to measure magnetic
field effects of proton dose distributions: simulation
study
S. Schellhammer
1,2
, B. Oborn
3,4
, A. Lühr
1,2,5
, S. Gantz
1,2
,
P. Wohlfahrt
1,2
, M. Bussmann
6
, A. Hoffmann
1,2,7
1
Helmholtz-Zentrum Dresden-Rossendorf, Institute of
Radiooncology, Dresden, Germany
2
OncoRay - National Center for Radiation Research and
Oncology, Medical Radiation Physics, Dresden, Germany
3
Wollongong Hospital, Illawarra Cancer Care Centre,
Wollongong, Australia
4
University of Wollongong, Centre for Medical Radiation
Physics, Wollongong, Australia
5
German Cancer Consortium DKTK, Partner Site Dresden,
Dresden, Germany
6
Helmholtz-Zentrum Dresden-Rossendorf, Institute of
Radiation Physics, Dresden, Germany
7
Faculty of Medicine and University Hospital Carl Gustav
Carus at the Technische Universität Dresden,
Department of Radiation Oncology, Dresden, Germany
Purpose or Objective
As a first step towards proof-of-concept for MR-integrated
proton therapy, the dose deposited by a slowing down
proton pencil beam in tissue-equivalent material is
assessed within a realistic magnet assembly. Furthermore,
radiation-induced activation and demagnetization effects
of the magnet are studied.
Material and Methods
The dose distributions of proton pencil beams (energy
range 70-180 MeV) passing through a transverse magnetic
field of a permanent C-shaped NdFeB dipole magnet
(maximum magnetic flux density B
max
= 0.95 T) while being
stopped inside a tissue-equivalent slab phantom of PMMA
were simulated (Figure 1). The beam was collimated to a
diameter of 10 mm. A radiochromic EBT3 film dosimeter
was placed centrally between the two phantom slabs
parallel to the beam’s central axis. 3D magnetic field data
was calculated using finite-element modelling (COMSOL
Multiphysics) and experimentally validated using Hall-
probe based magnetometry. A Monte Carlo model was
designed using the simulation toolkit Geant4.10.2.p02 and
validated by reference measurements of depth-dose
distributions and beam profiles obtained with Giraffe and
Lynx detectors (IBA Dosimetry), respectively. The beam
trajectory and lateral deflection were extracted from the
film’s planar dose distribution. Demagnetization was
assessed by calculating the dose deposited in the magnet
elements, and by relating this to radiation hardness data
from literature. A worst-case estimate of the
radioactivation of the magnet was obtained by taking into
account the most common produced mother nuclides and
their corresponding daughter nuclides.
Figure 1
: Simulation geometry.
Results
The Monte Carlo model showed excellent agr eement with
the reference measurements (mean absolute range
difference below 0.2 mm). The predicted planar dose
distribution clearly showed the magnetic fi eld induced
beam deflection (Figure 2). The estimated in-plane
deflection of the Bragg peak ranged from 0 cm for 70 MeV
to 1 cm for 180 MeV in comparison to no magnetic field.
No out-of-plane beam deflection was observed. Exposing
the film to 2 Gy at the Bragg peak was estimated to cause
a mean dose to the magnets of 20 µGy, which is expected
to produce negligible magnetic flux loss. The initial
activation was estimated to be below 25 kBq.
Figure 2
: Simulated dose distribution of a deflected
proton beam (180 MeV, 10
7
primary particles) on a film
dosimeter.
Conclusion
A first experimental setup capable of measuring the
trajectory of a proton pencil beam slowing down in a
tissue-equivalent material within a realistic magnetic field
has been designed and built. Monte Carlo simulations of
the design show that magnetic field induced lateral beam
deflections are measurable at the energies studied and
radiation-induced magnet damage is expected to be
manageable. These results have been validated by
irradiation experiments, as reported in a separate
abstract.
OC-0344 Experimental validation of TOPAS neutron
dose for normal tissue dosimetry in proton therapy
patients
G. Kuzmin
1
, A. Thompson
2
, M. Mille
1
, C. Lee
1
1
National Cancer Institute, Division of Cancer
Epidemiology and Genetics, Rockville, USA
2
National Institute of Standards and Technology,
Radiation Physics Division, Gaithersburg, USA
Purpose or Objective
In the last several years, the popularity and use of proton
therapy has been increasing due to its promise of a
dosimetric advantage over conventional photon therapy.
This is especially of great importance in pediatric patients
who have a higher risk of developing late effects. During
proton therapy 90% of scatter dose is from neutrons, which
can travel out of the treatment field and can be highly
biologically effective. In order to conduct epidemiological
investigations of the risk of long term adverse health
effect in proton therapy patients, it is imperative to
accurately assess radiation dose to normal tissue. Tool for
Particle Simulation (TOPAS) based on the GEANT4
Simulation Toolkit may be a computational option for
normal tissue dosimetry to support large scale
epidemiological investigations of proton therapy patients.
While previous works have benchmarked TOPAS for proton
dosimetry within treatment fields, there is a lack of
validation for neutron scatter and energy spectrum. In the
current study, we measured the energy spectrum of
scattered neutrons using a simple physical phantom
coupled with a series of Bubble Detectors irradiated by
Californium-252 neutron source.
Material and Methods