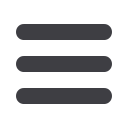

S241
ESTRO 36 2017
_______________________________________________________________________________________________
Purpose or Objective
Advances in intracranial stereotactic radiotherapy have
led to high gradient dose between tumor and normal tissue
and to dramatically reduced Planning Target Volume (PTV)
margins. Accurate definition of the gross tumor volume
(GTV) for stereotactic radiotherapy of brain metastases is
an essential key for the treatment planning. However, its
underestimation due to tumor growth during the delay
between planning and stereotactic radiotherapy may lead
to treatment failure.
Our purpose was to evaluate the tumor growth kinetics
and its impact during the delay before treatment of brain
metastasis secondary to lung cancer (LC) or melanoma
(ML).
Material and Methods
This retrospective monocentric study included all
consecutive patients (pts) treated for brain metastases
secondary to LC or ML between June 2015 and May 2016.
Margins from GTV to PTV were 2 mm. Imaging at diagnosis
of brain metastasis and preplanning imaging were
compared; GTV corresponding to the contrast
enhancement was analyzed. Linear extrapolation was used
to determinate the n minimum theoretical time leading
the diameter of the tumor to increase more than 4 mm
(T4mm).
Results
Out of 103 pts treated for brain metastasis by stereotactic
radiotherapy, 50 were treated for metastases secondary
to LC (n=26) or ML (n=24). Six pts were excluded because
of lack of imaging data. Median age was 68 years old
(range: 25-92). RPA status was 1 for 1 patient (2%), 2 for
33 pts (79%) and 3 for 8 pts (19%). Systemic treatment was
given at diagnosis for 19 pts (45 %). Radiotherapy was
delivered according to a monofraction scheme for 8 pts (3
LC and 5 ML metastasis), 3-fraction scheme (23 LC, 18 ML)
or 5-fraction scheme (2 LC, 3 ML).
A hundred and eight brain imaging (84 MRI, 24 CT-scan)
were analyzed. Comparison of imaging at diagnosis and
preplanning treatment showed bleeding inside metastasis
for one patient with primary LC; increased tumor volume
for 40 pts (ML n=25 ; LC n=15) ; stability for 11 pts (ML n=1
; LC n=10) and decreased volume for one LC patient.
Median delay between brain imaging at diagnosis and
pretreatment planning were: 28 days (range 8-107) for ML
pts and 31.5 days (range 7-70) for LC pts. Median Volumes
of GTV at diagnosis were 0.5 cm3 (range 0.05-8.6cm
3
) for
ML pts and 0.45cm
3
(range 0.05-6.1cm
3
) for LC pts; median
volumes of preplanning treatment GTV were 1.55 cm
3
(range: 0.2-9.9cm
3
) for ML pts and 0.85 (range 0.2-
10.4cm
3
) for LC patients. Linear extrapolation revealed a
median increase of tumor volume of 0.16 cm3/wk (range
0-0.8 cm3/wk) for ML and 0.06 cm3/wk (range 0-0.5
cm3/wk) for LC. Shorter T4mm was 15 days for ML patients
and 17 days for LC pts.
Conclusion
Maximal delay for treatment appeared to be 15 days for
ML patients and 17 days for LC patients to ensure that
tumor radius has grown less than to 2 mm. Above this
delay, clinicians should reconsider planning of treatment.
PV-0458 FMECA of Cyberknife process: two years’
experience for improvement
S. Cucchiaro
1
, D. Dechambre
1
, T. Massoz
1
, N. Gourmet
1
,
D. Boga
1
, N. Jansen
1
, P. Coucke
1
, M. Delgaudine
2
1
C.H.U. - Sart Tilman, Radiotherapy Departement, Liège,
Belgium
2
C.H.U. - Sart Tilman, STA Quality Departement, Liège,
Belgium
Purpose or Objective
Failure Modes Effects and Criticality Analysis (FMECA) is a
risk analysis allowing the identification of causes and
effects of a potential problem and the prioritization of
actions that can reduce this dysfunction. Our Radiation
Therapy Department used the FMECA as a strategy tool to
continuously improve treatment quality and safety. This
FMECA approach was applied to our Cyberknife (CK)
workflow process.
Material and Methods
Using the FMECA methodology, the CK workflow process
was defined with a flow chart and responsibility map
including a description of every step of prescription,
treatment preparation and treatment delivery. The
identification of possible risks was then carried out with
their origins and consequences. The evaluation was based
on 3 criteria: Severity (S), frequency of Occurrence (O)
and probability of Detection (D). Finally, we calculated
the Criticality Index (CI = S x O x D) for each of the
identified risks. The rating for each criterion is based on a
scale from 1 to 4. The Criticality Index can span a range
of 1 to 64.
Results
We defined 10 stages, with corresponding failure modes
presented in a table. At each stage, identified failures
with possible causes and consequences are listed and the
risk level assessed. A detailed scoreboard was obtained
presenting the risks and enabling easier identification of
priority actions to be undertaken. The board showed 66
possible failure modes. 8 of the top-ranked failure modes
were considered for process improvements. We also
crossed the scoreboard obtained with the adverse events
most often reported on 2015. We found 2 correspondences
between failure modes and adverse events reported. We
therefore also considered that in the implementation of
preventive/improvement actions to take. A review of this
analysis was done in September 2016. Therefore, at this
moment, a revaluation of the process, failures, ratings and
implemented actions was performed with each members
of the CK team. The correlation with reported adverse
events was also made. We had one failure mode that has
to be changed from a moderate to an unacceptable level
because an incident was reported following a non-update
procedure. New improvement actions have been
implemented directly. In order to continue our proactive
approach to risk analysis a systematic annual review of this
analysis is now introduced in routine. All this, in relation
to the reported adverse events. The figure shows an
extract of the FMECA scoreboard obtained for CT
simulation
and
contouring
stage.