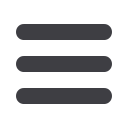

S282
ESTRO 36 2017
_______________________________________________________________________________________________
Purpose or Objective
IROC Houston’s head and neck phantom has been used to
verify IMRT dose delivery for over a decade. While the
passing rate has seen gradual improvement, at present
more than 10% of institution’s that irradiate this phantom
still fail to meet the generous acceptability criteria. This
study explores the causes of these failures.
Material and Methods
To pass the head and neck phantom, the TPS-calculated
dose must agree within 7% with TLD measurement at 6
assessed locations in two PTVs. Two film planes must show
>85% of pixels passing a 7%/4mm gamma criterion. Failed
irradiations over the past year were evaluated
qualitatively for the cause of the failure: positioning
errors, systematic dosimetric errors, and other errors.
Based on the finding that most errors were systematic
dosimetric errors, further quantitative exploration was
done. Potential errors in institutional TPS beam models
were evaluated using an independent recalculation of the
phantom’s treatment plan. This was done using
“reference” beam models constructed from aggregated
IROC Houston measurements for different classes of
accelerator that were developed in Mobius 3D. 259
phantom plans were recalculated with these models.
Results
On qualitative evaluation, only 13% of failures were
attributed to localization errors, 18% were other non-
systematic errors, and the vast majority, 69%, were
systematic dosimetric errors: the dose distribution had the
right shape and was in the right place, but it was the
wrong magnitude. The independent recalculation of 259
phantom plans showed many cases where our reference
model was less accurate than the institution, but a
shocking number of cases where our recalculation was
more accurate, both significantly (based on a 2-sided t-
test with a failure detection rate correction applied), and
substantially (>2% average improvement across the 6 TLD).
The independent recalculation was significantly and
substantial better than the institution’s calculation in 18%
of all cases, and in 68% of cases where the institution
failed the phantom.
Conclusion
Failures of the IROC Houston IMRT phantom
overwhelmingly indicated a deficiency in the beam model.
This is concerning because this beam model is applied to
all patients, suggesting suboptimal treatment at nearly 1
in 5 radiotherapy facilities. It also indicates that physicists
should increase attention to beam modeling.
OC-0537 A remote EPID-based dosimetric auditing
method for VMAT delivery using a digital phantom
concept
P. Greer
1
, K. Legge
2
, N. Miri
2
, P. Vial
3
, T. Fuangrod
1
, J.
Lehmann
1
1
Calvary Mater Newcastle Hospital, Department of
Medical Physics, Newcastle, Australia
2
University of Newcastle, School of Physical and
Mathematical Sciences, Newcastle, Australia
3
Liverpool Hospital, Radiation Oncology, Sydney,
Australia
Purpose or Objective
Current methods to perform dosimetric audits for
participation in clinical trials are expensive and time-
consuming with high failure rates. Currently nearly 2/3 of
trial centres in Australia/New Zealand have not had an
independent VMAT dosimetric audit. The aim of this work
is to develop an inexpensive new method for remote
dosimetric VMAT auditing.
Material and Methods
Remote centres are provided with CT datasets and
planning guidelines to produce trial VMAT plans for a head
and neck and a post-prostatectomy treatment. The plans
are transferred in the treatment planning system (TPS) to
two digital water equivalent phantoms, one cylindrical (20
cm diameter) and one flat phantom. EPID cine images are
then acquired during the VMAT delivery to the EPID panel
in air. The cine images are backprojected to 3D dose in
the digital cylindrical phantom using an established
conversion method. This dose is then compared to TPS
dose at a central site. Individual 2D arc doses (with gantry
angles collapsed to zero in the TPS) are compared to EPID
derived dose at 10 cm depth in the flat phantom. For
Varian systems EPID images are obtained for Clinac
systems using cine imaging mode and for Truebeam
systems using image processing service which stores
individual cumulative frames. Both of these systems store
the gantry angle for the image in the header. For Elekta
systems the service XIS software was used as the clinical
cine mode normalises every image. This software acquires
individual frames without a gantry angle. Three methods
for gantry angle measurement were investigated, an
inclinometer, a video-based method, and the machine
iCom log files. The video-based method uses a printed
green arrow attached to the gantry, with a mobile phone
video recording during delivery. Colour detection and
image processing were used to determine the angle in
each movie frame.
Results
To date six Varian centres have been fully analysed. The
3D gamma comparison results (3%,3mm for >10% of global
maximum dose) were greater than 95% pass-rate for five
centres and 93% for the one centre. For the 2D individual
arcs all results were greater than 95% pass-rate for
3%,2mm criteria. Three Elekta centres have had
preliminary investigations. Gantry angle comparisons show
that the video method is comparable to the inclinometer
and is easy to perform using only a printed piece of paper
and mobile phone. Challenges for the method include
synchronisation of the angle measurement and the EPID
frames, and difficulty of use of the XIS software.
Figure 1. Comparison of gantry angles recorded for Elekta
system, inclinometer (NG360), iCom log file and mobile
phone method (insert)
Figure 2. Comparison of EPID derived dose and TPS dose
calculation for a Varian Truebeam system (sagittal dose
plane)