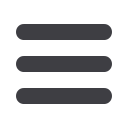

S423
ESTRO 36 2017
_______________________________________________________________________________________________
Gesellschaft für Schwerionenforschung (GSI) were used as
reference data.
Results
For all physics lists, the relative dose differences up to the
Bragg peak were found to be less than 4% compared to
measurements. Beyond the Bragg peak, in the so-called
fragmentation tail, differences increased notably, by up
to one order of magnitude. However, the absolute dose
difference in the fragmentation tail was comparable to
the absolute difference before the Bragg peak. The QMD
model systematically overestimated whereas the other
models underestimated the dose in the fragmentation tail.
Overall, deviations to the measurement were less than 2%
of the maximum dose for all models, disregarding the dose
fall off region due to the steep dose gradient.
Partial charge changing cross sections simulated with the
BIC, BERT and QBBC models deviated up to 60% from the
measurements, INCLXX up to 38% and the QMD model up
to 24%. However, the significance on fragmentation in
particle therapy is limited by the high energy equal to 630
MeV/u used in the measurements.
Conclusion
IDDs simulated with Gate/Geant4 agreed well with
measurements for all models under investigation,
although notable deviations were observed in the
fragmentation tail. Measured partial charge changing
cross sections could best be reproduced using the QMD
model, whereas the BIC model showed considerable
discrepancies. Therefore, Gate/Geant4 can be considered
a valid dose calculation tool for oxygen ion beams and will
further on be used for the development of a pencil beam
algorithm for oxygen ions. The QMD model is
recommended in order to obtain accurate fragmentation
results, which is essential for radiation oncology purposes.
PO-0802 Experimental validation of single detector
proton radiography with scanning beams
C. Chirvase
1
, K. Teo
2
, R. Barlow
1
, E.H. Bentefour
3
1
International Institute for Accelerator Applications, The
University of Huddersfield, Huddersfield, United
Kingdom
2
University of Pennsylvania, Department of Radiation
Oncology, Philadelphia PA, USA
3
Advanced Technology Group, Ion Beam Applications
s.a., Louvain-la-Neuve, Belgium
Purpose or Objective
Proton radiography represents a potential solution to solve
the uncertainties of dose delivery in proton therapy. It can
be used for in-vivo beam range verification; patient
specific Hounsfield unit (HU) to relative stopping power
calibration and improving patient set-up. The purpose of
this study is to experimentally validate the concept of the
energy resolved dose measurement for proton radiography
using a single detector with Pencil Beam Scanning (PBS).
Material and Methods
A 45 layers imaging field with a size of 30 x 30 cm
2
and
energies between 226 MeV and 115 MeV is used to deliver
a uniform dose. The dose per spot is 4.25 mGy with spot
spacing equal to the beam sigma. The imaging field is first
delivered on wedge shaped water phantom to produce
calibration library of Energy Resolved Dose Functions
(ERDF) between 0 cm and 30 cm. Then, the same imaging
field is delivered in three different configurations: a stack
of solid water in a stairs shape with thicknesses between
1 mm and 10 mm – that determines the accuracy with
which the WEPL (water-equivalent path length) can be
retrieved, CIRS lung phantom – that illustrates the
accuracy on the density of multiple materials and a head
phantom – which represents a realistic case of
heterogeneous target.
As shown in Figure 1, proton radiographs are recorded with
a commercial 2D detector (Lynx, IBA-Dosimetry,
Schwarzenbruck, Germany) which has an active area of
300 × 300 mm² with an effective resolution of 0.5 mm.
Figure 1. Experimental set-up with an example of proton
radiograph imaged at beam energy of 220 MeV.
Results
In this study we demonstrate the robustness of the energy
resolved dose measurement method for single detector
proton imaging. It shows the capability to determine the
WEPL with sub-millimeter accuracy in a homogeneous
target and performs well in heterogeneous target, proving
an accuracy better than 2 mm even in most heterogeneous
areas of a head phantom. These performances are
achieved by using an imaging field with as little as 5
energy layers with spacing up to 10 mm between the
layers.
Although the optimization of the imaging dose was not a
goal of this study, only ~21 mGy per cm
2
is sufficient to
obtain the above accuracies. This dose can be further
decreased by using a detector with higher sensitivity and
by reducing the number of beam spots per layer of the
imaging field.
Conclusion
Proton radiography with single detector using energy
resolved dose measurement did show potential for clinical
use. Further studies are needed to optimize the imaging
dose and the clinical workflow.
PO-0803 CloudMC, a Cloud Computing application for
fast Monte Carlo treatment verification
H. Miras
1
, R. Jiménez
2
, R. Arrans
1
, A. Perales
3
, M. Cortés-
Giraldo
3
, A. Ortiz
1
, J. Macías
1
1
Hospital Universitario Virgen Macarena, Medical Physics,
Sevilla, Spain
2
Icinetic TIC SL, R&D division, Sevilla, Spain
3
Universidad de Sevilla, Atomic- Molecular and Nuclear
Physics Department, Sevilla, Spain
Purpose or Objective
CloudMC is a cloud-based solution developed for r educing
time of Monte Carlo (MC) simulation s through
parallelization in multiple virtual computing nodes in the
Microsoft’s cloud. This work presents an update for
performing MC calculation of complete RT treatments in
an easy, fast and cheap way.
Material and Methods
The application CloudMC, presented in previous works, has
been updated with a solution for automatically perform
MC treatment verification. CloudMC architecture (figure
1) is divided into two units. The processing unit consists of
a web role that hosts the user interface and is responsible
of provisioning the computing worker roles pool, where
the tasks are distributed and executed, and a reducer
worker role that merges the outputs. The storage unit
contains the user files, a data base with the users and
simulations metadata and a system of message queues to
maintain asynchronous communication between the front-
end and the back-end of the application.