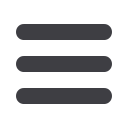

S487
ESTRO 36 2017
_______________________________________________________________________________________________
adjacent contours, 3) conformity index (CI) between
adjacent contours, 4) presence of air or bone across the
line of the contour, 5) presence of air or bone within 5 mm
outside of the contour boundary, and 6) presence of
spacing > 20 mm between adjacent contour points.
The threshold values for the metrics 1-3 were calculated
from the rectum contours drawn by oncology experts on
315 pelvic kVCT scans, where we used 6 mm superior-
inferior contour spacing to match the slice spacing of the
IG scans. The settings for metrics 4-6 were determined
empirically. Our software developed in Python 2.7
analysed the DICOM RTSTRUCT and IG scan data, applied
the metrics and recorded the evaluation results in a
spreadsheet. A contour was marked as “error” if any of
the thresholds defined in the metrics was triggered.
Results
The automatic evaluation of 11519 contours for 33
patients took 6 minutes on a computer with 8 GB RAM and
1.6 GHz Intel Xeon CPU. The evaluation results were
compared to the errors recorded by a human observer, and
confusion matrices were calculated. The mean error
prevalence in the observer evaluation was 0.29 ± 0.1. Our
algorithm achieved a mean sensitivity of 0.84 ± 0.1 (range
[0.58 – 1.0]) and a mean specificity of 0.88 ± 0.1 (range
[0.51 – 1.0]). One patient data set totalling 339 slices was
evaluated with a sensitivity and specificity of 1.0.
Conclusion
Metric-based evaluation of rectum contours is a feasible
alternative to evaluation of contours by a human observer.
It provides an unbiased contour classification and detects
over 80% of typical errors in the contours. The method can
be used to assess the performance of automated
contouring tools and to aid the development of improved
contouring software.
PO-0893 Improving CBCT image quality for daily image
guidance of patients with head/neck and prostate
cancer
I. Chetty
1
, P. Paysan
2
, F. Siddiqui
1
, M. Weihua
1
, M. Brehm
2
,
P. Messmer
2
, A. Maslowski
3
, A. Wang
3
, D. Seghers
2
, P.
Munro
2
1
Henry Ford Health System, Radiation Oncology, Detroit,
USA
2
Varian Medical Systems Imaging Laboratory GmbH, Image
Enhancement and Reconstruction, Baden-Daettwil,
Switzerland
3
Varian Medical Systems- Inc., Oncology Systems, Palo
Alto, USA
Purpose or Objective
Image quality of on-board CBCT imaging in radiation
therapy generally falls short of diagnostic CT in particular
in terms of low contrast visibility. This limits the
application of CBCT mainly to patient setup based on high
contrast structures. We address these limitations by
applying advanced preprocessing and reconstruction
algorithms to improve patient setup and facilitate
advanced applications like adaptive radiotherapy.
Material and Methods
The
commercially
available
TrueBeam
CBCT
reconstruction pipeline removes scatter usi ng a kernel-
based correction followed by filtered bac k-projection-
based reconstruction (FDK). These reconstruction n
pipeline steps are replaced by a physics-based scatter
correction (pelvis only) and an iterative reconstruction.
We use statistical reconstruction that takes the Poisson
distribution of quantum noise into account, an d applies
an edge preserving image regularization. The advanced
scatter correction is based on a finite-ele ment solver
(AcurosCTS) to model the behavior of photons as they pass
(and scatter) through the object. Both algorit hms have
been implemented on a GPU cluster pla tform, and
algorithmic acceleration techniques are utilized to
achieve clinically acceptable reconstruction times. The
image quality improvements have been an alyzed on
TrueBeam kV imaging system phantom scans, as well as
on daily CBCT scans of head/neck and prostate cancer
patients acquired for image-guided localization.
Results
Artifacts in head/neck FDK reconstructions (Fig . 1) e.g.
resulting from photon starvation in the shoulder region or
cone-beam are highly reduced in the iterative
reconstructions. The iterative reconstruction s show
enhanced soft tissue definition providing better cl arity for
boundary definition (see the level 2 lymph node located in
the contoured region of the axial view, Fig. 1). The
advanced scatter correction applied for pelvis scans
removes residual scatter artifacts, increasing the mean
homogeneity from 78.2 HU ± 18.0 HU to 20.9 HU ± 10.9 HU
within the bladder region of 9 daily CBCT scans of typical
prostate patients. Iterative reconstruction provides
further benefit by reducing image noise as well as
eliminating streak and cone-beam artifacts, thereby
significantly improving soft-tissue visualization, as noted
in the clinical pelvis CBCT scan (Fig. 2). The noise level
was reduced to 45% of the original value.
Conclusion
Statistical reconstruction in combination with advanced
scatter correction substantially improves CBCT image
quality by enabling removal of artifacts caused by
remaining scatter, projection noise, photon starvation,
and cone-beam angle. These artifact reductions improve
soft tissue definition that is necessary for accurate
visualization, contouring, dose calculation, and
deformable image registration in clinical practice. The
presented improvements are expected to facilitate soft
tissue-based patient setup. Promise has been
demonstrated for new applications, such as adaptive