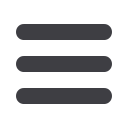

S879
ESTRO 36 2017
_______________________________________________________________________________________________
This can potentially minimize operator dependence or
even remove the need of the skilled operator.
Material and Methods
In this study, co-registered CT and 3D transperineal US
(TPUS) volumes with corresponding anatomical structure
delineations of a prostate cancer patient were available.
After preprocessing, a thresholding-based segmentation
algorithm was used to extract the bones from the CT scan
(Fig. 1A). The retrieved bone mask and the internal
perineum boundaries enabled the identification of the
patients’ perineal area on the skin (Fig. 1A-B-C).
Subsequently, the scrotum was localized in order to
identify the underlying perineal skin. Finally, the areas on
which the US probe could not be positioned in clinical
practice (e.g. the region around the anus) were removed.
In this way, a skin area accessible for TPUS volume
acquisition was automatically identified (Fig. 1D).
All probe setups proposed by the algorithm should allow
visualization of the whole prostate and seminal vesicles,
as well as the adjacent edges of bladder and rectum, as
clinically required. To determine these setups, the
accessible skin area, in combination with the structure
delineations and an estimation of the potential probe
pressure, was used. The setup that also allowed
visualization of most of the remaining anatomical
structures was considered the best option.
Results
By positioning a virtual probe on the patients’ virtual skin
and subsequently translating it along the Y axis (1 mm
step), rotating around Y or Z axes (up to ±3 degrees with
1 degree step) or rotating around the X axis (up to ±15
degrees with 3 degree step), 12,936 possible probe setups
were identified. In total 108 of these setups allowed
visualization of all clinically required structures without
the occurrence of blockage by the patients’ bones.
In Fig. 2 the sector of the best probe setup is superimposed
in yellow on the center slices of the patients’ CT volume.
If the physician had been provided with this setup prior to
the US scan, potentially 96% of the delineated anatomical
structures could have been visualized, in comparison with
87% using the current setup (cyan sector in Fig. 2), which
was manually determined by trial-and-error.
Conclusion
The best probe setup out of 108 possible setups could
potentially increase the visualization of the anatomical
structures from 87% to 96%, while still fulfilling the clinical
requirements. Future steps should focus on enabling
skilled and unskilled workers to position the US probe
according to the calculated setup.
EP-1643 Simulate baseline shift uncertainties to
improve robustness of proton therapy treatments
K. Souris
1
, A. Barragan
1
, D. Di Perri
1
, X. Geets
1
, E.
Sterpin
1
, J.A. Lee
1
1
UCL - IREC Molecular Imaging Radiology and Oncology
MIRO, Molecular Imaging Radiology and Oncology MIRO,
Brussels, Belgium
Purpose or Objective
Several studies reported both systematic and random
variations of the mean position of mobile tumors from
fraction to fraction. This so-called baseline shift is a major
source of uncertainties for mobile targets and can
jeopardize treatment quality. Unlike conventional photon
therapy, the inclusion of this error in a PTV margin is
inadequate in proton therapy because of the range
uncertainties. Accounting for this uncertainty in a robust
optimizer is much more appropriate, using for instance
population-based estimations of the shifts. We developed
a baseline-shift model able to automatically generate
modified 4D-CT series used as uncertainty scenarios in the
TPS.
Material and Methods
An average CT scan and a Mid-Position CT scan (MidPCT)
of the patient at planning time are generated from a 4D-
CT data. The GTV contour in the MidPCT represents the
mean position of the tumor along the breathing cycle. Our
model can simulate a baseline shift by generating a local
deformation field that moves the tumor on all phases of
the 4D-CT, without creating any non-physical artifact. The
deformation field is comprised of normal and tangential
components with respect to the lung wall, in order to
allow the tumor to slide within the lung instead of
deforming the lung surface. The deformation field is
eventually smoothed in order to enforce continuity. Two
4D-CT series acquired at 1 week of interval were used to
validate the model.
Results
After rigid registration, a baseline shift of 9.5 mm is
measured between the first- and second-week 4D-CT sets
(W1-CT and W2-CT). In order to validate our model, a third
4D-CT series (BS-W1-CT) was generated from W1-CT to
reproduce the measured shift (Figure 1). Water equivalent
thickness (WET) has been computed for each voxel of the
3 MidPCTs and revealed that the baseline shift between
W1-CT and W2-CT led to a root mean square error (RMSE)
of 0.52 mm in the GTV. This WET RMSE was reduced to
0.18 mm between W2-CT and the simulated BS-W1-CT. In
addition, a proton therapy plan was optimized on the
average W1-CT scan and recomputed on the average W2-
CT and BS-W1-CT scans. Figure 2 compares the resulting
DVH for all dose distributions. The dose distribution
computed on BS-W1-CT reproduces the dose degradation