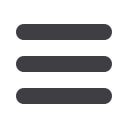

one-dimensional (1D) sliding. This is akin to joining a book
club to sample a few dozen of its members in search of a po-
tential mate. The filament around broken DNA can bind to a
random DNA sequence but slides along the DNA back and
forth over hundreds of basepairs. This local search can be
much more effective when combined with a three-dimen-
sional search. If the local search is unsuccessful, the fila-
ment can dissociate and bind another region of DNA,
which would be equivalent to joining a different club,
such as a knitting club.
Using FRET, one can probe for such 1D sliding activity.
A green dye on the filament and a red dye on the target DNA
would result in fluctuations in FRET, anticorrelated changes
in the intensities of green and red signals, providing evi-
dence for 1D sliding
( 6). But can the filament really find
the target sequence through sliding? To address this ques-
tion, we can put two near-matching sequences on the target
DNA. The filament would spend some time on one near-
matching sequence and after it realizes that the match is
not perfect, it will leave and slide, and will land on the other
near-matching sequence, and this can continue back and
forth. This would be like dating two twin brothers, each of
whom is not a perfect match. Overall, single-molecule
FRET measurements suggest that such 1D sliding may
accelerate the finding of the matching sequence by as
much as 250 times, possibly aiding DNA repair greatly.
Marriage of single-molecule FRET and optical
tweezers
Another single-molecule technique, called optical tweezers,
or what I call ‘‘chopsticks made of light,’’ can apply very
small forces, down to 10
12
N of force, and measure the
response mechanically at the nanometer level. For example,
optical tweezers have been used to measure the step size of
many molecular motors
( 2). Recently, the precision of opti-
cal tweezers has improved to the angstrom scale, almost the
size of a water molecule
( 7).
In optical-tweezers measurements, it is as if you are clos-
ing your eyes and using your hands to manipulate and mea-
sure the response of an object, whereas in fluorescence
measurements, you have your hands tied in back and
make passive observations with your eyes. By combining
the two, we can hope to sample the best of both worlds.
For example, we can use optical tweezers to measure the
activities of single proteins such as helicases, which unwind
DNA into single strands using the energy of ATP molecules
and at the same time measure the conformational changes of
the protein using FRET. Using such a hybrid instrument, it
was shown that a helicase called UvrD unwinds DNA
when it takes the ‘‘closed’’ conformation and rezips DNA
when it takes the ‘‘open’’ conformation
( 8). When a related
helicase was forced to maintain the closed form, it became a
superhelicase that can unwind thousands of basepairs
without falling off, even against a very strong opposing
force
( 9). This superhelicase may be useful for various
biotechnological amplifications, such as rapid pathogenic
DNA detection and sequencing in developing countries or
during surgery in hospitals.
Future outlook
Single-molecule measurements have revealed the amazingly
complex but elegant abilities of nature’s nanomachines to
FIGURE 1 How to record the dancing moves of
a rap musician. Labeling the right hand with a
green dye and the left hand with a red dye makes
it possible to follow the distance changes between
the two hands as a function of time. When the
two hands are very close to each other (horseback
posture), fluorescence excitation energy is trans-
ferred from the green dye to the red dye, in a pro-
cess called FRET, so that the red signal is
stronger than the green. When the two hands are
far away from each other (cowboy), there is little
energy transfer and the green signal is stronger.
Once the dance finishes and the red dye is released
from the musician, only green signal is observed. In
the case of protein nanomachines, FRET occurs
over a length scale of a few nanometers. Because
of close proximity, the two dyes would not appear
as two separate objects in single-molecule imaging.
Instead, one sees a single object that changes color
over time. This illustration was created by Dr. Jin-
gyi Fei at the University of Chicago. To see this
figure in color, go online.
Biophysical Journal 110(5) 1004–1007
1006
Ha