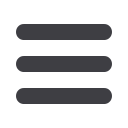

S416
ESTRO 36
_______________________________________________________________________________________________
detectors. A rotational symmetric Gaussian horizontal
beam profile and exponential decaying depth dose profile
in the vicinity of the pellet was fitted to the measured
profiles. Both 6 MV and 6 MV FFF beams were considered.
Results
The fit of the beam profile in three dimensions was based
on two parameters: the variance for the Gaussian profile
and the gradient of the depth profile. The parameters in
turn were both changing as function of FS. Using the fitted
beam profiles, an analytical model was developed for the
calculation of volume correction factors k
V
for given FS
(see Table 1).
Table 1: Calculated volume correction factors k
V
,
temperature and volume corrected output factors (OF)
with SD being one standard deviation (SD) are displayed
for the 6 MV and 6 MV FFF beams as function of the field
size FS.
Conclusion
Volume averaging was found to influence the alanine
measurements by up to 6 % for the smallest field size. For
a cylindrical detector irradiated along the symmetry axis
of the detector, simple analytical expressions of the
volume correction factors were obtained. The analytical
expression gives valuable insight in the volume correction
factor k
V
as function of field size and the radius of the
sensitive volume of the detector. The method presented
here would be applicable for other detectors. With a
defined geometry of the sensitive volume of the detector
relative to the central axis of the beam the volume
correction factor can either be calculated analytically or
numerically as function of FS.
Poster: Physics track: Dose measurement and dose
calculation
PO-0785 A pencil beam algorithm for protons including
magnetic fields effects
F. Padilla
1
, H. Fuchs
1
, D. Georg
1
1
Medizinische Universität Wien Medical University of
Vienna, Department of Radiation Oncology, Vienna,
Austria
Purpose or Objective
Magnetic Resonance Image (MRI) has the potential to
increase the accuracy and effectiveness of proton
therapy. Previous studies on that topic demonstrated that
corrections in dose calculation algorithms are strictly
required to account for the dosimetric effects induced by
external magnetic fields. So far, a real dose calculation
possibility including a trajectory corrected approach was
missing. In this study, we developed a pencil beam
algorithm (PBA) for dose calculation of a proton beam in
magnetic fields.
Material and Methods
MC simulations using the GATE 7.1 toolkit were performed
to generate first benchmarking data and subsequent
validation data for the PBA. The PBA was based on the
theory of fluence weighted elemental kernels. A novel and
non-symmetric exponential tailed Gauss fitting function
was used to describe the lateral energy deposition profiles
in water. Nuclear corrections, multiple scattering and
charged particle drifting were accounted by means of a
look-up table (LUT) approach. Longitudinal dose
depositions were estimated from the LUT and corrected
using a water-equivalent depth scaling. In a first step
proton beams in the clinical required energy range 60 –
250 MeV with transverse external magnetic fields ranging
from 0 – 3T were analyzed in a 40x40x40 cm
3
water
phantom. Next validation simulations were performed for
different phantom configurations, e.g. using a simple
water box or slab-like geometries with inhomogeneities of
different materials and volumes. Percentage depth dose
curves (PDD) and two-dimensional dose distributions were
calculated to assess the performance of the PBA.
Results
For PDD in water discrepancies between the PBA and MC
of less than 1.5% were observed for all the depth values
before the Bragg-Peak (see Figure 1). An increasing value
of up to 6% was found in the distal energy falloff region,
where dose values represents around 1% of the maximum
dose deposition. In all cases, maximum range deviations
of the results were less than 0.2 mm. Deviations between
two dimensional dose maps obtained with PBA and GATE
remained below 1% for almost all the proton beam
trajectory, reaching a maximum value up to 4% in the
Bragg-Peak region, see Fig. 2. As expected, agreement
became worse for high energy protons and high intensity
magnetic fields.
Fig. 1. PDD curves comparing the PB algorithm with MC
simulations for proton beams in water. Relative
discrepancies are shown in the top region of the graph.
Fig. 2 Relative dose difference map for a 240 MeV proton
beam in water exposed to a 3T transverse field.
Conclusion
The proposed pencil beam algorithm for protons can
accurately account for dose distortion effects induced by
external magnetic fields. Corrections of dose distributions
using an analytical model allows to reduce dose