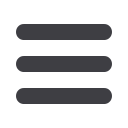

S961
ESTRO 36
_______________________________________________________________________________________________
All fields acquired in the study have 20x20 cm size, 5 MU
each. To obtain the RC of the EPID at 0º gantry, four fields
are used, at 0, 90, 270 and 180 º of collimator and 0º
gantry with a radiopaque crosshair attached to the LINAC
head. RC is calculated with two methods: using the
radiation field limits and with the radiopaque crosshair
center.
Second series of measures are acquired with a
BB
(bearing
ball) placed in laser isocenter and with a tray with four
smaller BB fixed in it, in the periphery of the field. Images
are obtained over a 360º arc, with 15 º gantry steps at 0º
collimator angle.
Cross reference of the 4 smaller BB positions with the RC
(determined in the first step) are made at 0º collimator
and 0º gantry. This gives to the 4 BB the ability to
determine RC position in subsequent gantry angles.
EPID sag is calculated for all gantry angles taking into
account the laser isocenter BB position in each EPID image
and compared with 0 º gantry angle. EPID + Gantry sag is
determined taking into account the mean position of the
BB fixed in the tray. The Gantry sag is obtained after
subtraction of EPID sag from EPID + Gantry sag.
Changes in SDD (Source-Detector Distance) are obtained
measuring the distance between two tray BB (d) and
comparing then to the distance (d
0
) for SDD for 0º gantry
angle (SDD
0
) in the way as Eq. reflects.
ΔSDD = SDD
0
· (d/d
0
-1)
A MATLAB in-house software is developed to make the
image analysis. The BBs and the center of radiopaque
crosshair is determined in each direction (in-plane and
cross-plane) with sub-pixel accuracy, 3 profiles near de BB
are obtained and fitted to Gaussian curves, the mean
maximum of the 3 curves is calculated. Radiation field
center is obtained calculating the 50% pixel value of a
vertical and horizontal profile displaced from the center
in case of BB in the image center.
Results
The LINAC measurements take no longer than 2 hours.
The RC for the EPID at 0º gantry obtained with radiopaque
crosshair is 1.11 and -1.02 mm for cross-plane and in-plane
directions, respectively. The RC using radiation field limits
is less than 0.3 mm away from this.
EPID RC is not plotted in Fig. 1 for clarity but is obtained
from the EPID + gantry sag measurements after adding the
RC for 0º gantry angle.
The major change in SDD is less than 1.4 cm (for 180º) from
SDD at 0º gantry angle (see Fig. 2).
Conclusion
The method presented is a useful, necessary and not too
time expending tool to characterize the EPID and Gantry
sag of a LINAC when EPID will be used in LINAC QA.
EP-1746 A new method for exact co-calibration of the
ExacTrac X-ray system and linac imaging isocenter
H.M.B. Sand
1
, K. Boye
2
, T.O. Kristensen
1
, D.T. Arp
1
, A.R.
Jakobsen
1
, M.S. Nielsen
1
, I. Jensen
1
, J. Nielsen
1
, H.J.
Hansen
1
, L.M. Olsen
1
1
Aalborg University Hospital, Department of Medical
Physics- Oncology, Aalborg, Denmark
2
Zealand University Hospital, Radiotherapy Department,
Næstved, Denmark
Purpose or Objective
To evaluate a new user independent sub-millimetre co-
calibration method between the X-ray isocenter of the
ExacTrac® (ET) system and the imaging isocenter of the
linear accelerator (linac).
Material and Methods
The new calibration method was evaluated on five linacs
from Varian, three Clinacs with the On Board Imager
system and two TrueBeams, all equipped with ET and
robotics from Brainlab. A BrainLAB isocenter calibration
phantom with five infrared markers attached on the top
and a centrally embedded 2 mm steel sphere was used in
the setup. Orthogonal MV-kV-image pairs of the
calibration phantom were acquired at the four quadrantal
gantry angles using the linac imaging system (LIS). In-
house made software detected the 2D position of the steel
sphere in each acquired image and from this determined
the 3D couch translation required to move the steel sphere
to the LIS isocenter. To accurately perform the
translation, we applied the sub-millimetre real-time
readout feature of the ET infrared system, which was set
to track the infrared markers of the phantom.
Subsequently, the origin of the ET system was calibrated
to match the optimal phantom position and hence the LIS
isocenter. Regular runs of the Varian IsoCal-routine
assured correspondence between the radiation isocenter
and
the LIS isocenter .
In the standard calibration method former used, the
calibration phantom was positioned based on one set of
MV-kV-images, manually interpreted by the user.
Results
The deviation between the ET X-ray isocenter and the LIS
isocenter was determined by evaluating the 3D deviation
vector for the new user independent optimal positioning
of the calibration phantom relative to the LIS isocenter. In
ten successive calibrations performed by different users in
a time period of nearly half a year, the 3D deviation vector
ranged from 0.03 mm to 0.10 mm with an average of 0.07
mm and a standard deviation (SD) of 0.02 mm.
Simultaneously, the 3D deviation vector was determined
for the standard calibration method, also in ten successive
calibrations and performed by different users. Here the 3D