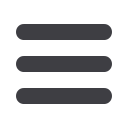

S103
ESTRO 36 2017
_______________________________________________________________________________________________
the wider spectrum of Health Technology Assessment
(HTA). Although more limited types of evaluations exist,
only the formal comparison of costs and effects between
two or more alternative interventions is considered to be
a full economic evaluation. To date, economic evaluation
has been mainly applied to pharmaceutical interventions.
Less attention has been paid to other types of
intervention, including those involving advanced health
technologies in radiotherapy. Overall, the fundamental
problem in determine the cost-effectiveness for new
technologies is the lack of valid data on effects as well as
costs. While randomized trials provide the most robust
clinical evidence of comparative efficacy, it is widely
recognized that high-level evidence is rarely achievable
with new technology, due to methodological and ethical
problems. Decision making in high technology areas is
therefore more challenging since adequate data is often
lacking. The number of published well performed
economic evaluations of radiotherapy using appears quite
low. As a consequence, there is limited robust evidence
on the effectiveness and cost effectiveness of
radiotherapy in cancer. Besides, it seems that results of
apparently similar cost-effectiveness studies are often not
comparable, since there is no uniformity on used input
variables – e.g. patient and tumor characteristics,
assumed impact of the treatment on outcome, treatment
costs – which is essential for the final result of the
analysis. Therefore, comparing results of for example
Markov model analysis were different variables are used
doesn’t make sense. Let’s take a closer look at the case
of proton therapy. It seems hard, or even impossible, to
estimate the cost-effectiveness of proton therapy based
on the published literature, mainly due to a lack of data.
This does not, however, relieve the many proton centers
that have recently become operational from the moral
duty to generate prospective evidence in terms of clinical
outcome and value for money. Even if they do, this will
take many years, whereas guidance in how to most
optimally allocate resources to these novel treatments is
urgently needed. Of course, more and better data, will
result in better outcome and more robust conclusions,
however this lack of data was already obvious ten years
ago, we might wonder if an adequate dataset will ever be
available. A model-based approach could be the solution
based on subgroup or individual patients. Applying NTCP
models forms the decisive link can generate evidence
regarding the value of proton therapy, and helps to create
enriched cohorts of patients who are likely to benefit from
protons. Next, it is possible to quantitatively assess the
effectiveness of proton therapy for individual patients,
comparing photon and proton treatments on dose metric,
toxicity and cost-effectiveness levels, retrieved from a
decision support system. Gathering good clinical and cost
data remains essential in defining the cost-effectiveness
of new technologies, such as proton therapy. In the
absence of level 1 evidence, well-performed modelling
studies taking the uncertainties, available cost and
outcome parameters into account, can help to tackle the
problem. Because it is evident that protons will not be
cost-effective for a total group of patients but for a subset
of patients, we shouldn’t look at the whole population
anymore but at an individual patient level. Well-designed
decision support systems will play an important role here.
Whereas agreement on used input variables and primary
endpoints remains still essential.
SP-0206 Tips and tricks for safe and effective routine
clinical application
F. Duprez
1
1
Universitair Ziekenhuis Gent, Radiotherapie-Oncologie,
Gent, Belgium
Since almost a decade, adaptive (ART) and multimodality
image guided radiotherapy (IGRT) have been investigated
with the aim of improving radiotherapy in many settings.
Several planning studies, observational studies and
prospective trials have demonstrated the feasibility and
theoretical advantages of ART and IGRT. However, the
implementation of ART and IGRT also imply incremental
work load, use of imaging techniques and extra or longer
hospital visits for patients that can already be
overwhelmed by standard procedures in their ill status.
Unlike the fact that there is yet no large-scale evidence of
the safety or cost effectiveness of these techniques in
clinical routine, dedicated hardware and software for ART
and IGRT are commercially available and implemented in
an increasing number of centers.
This lecture will focus on the clinician-oriented point of
view. ART might be planned before start of therapy, e.g.
consecutive plannings at certain timepoints in the
radiation course, while it might also be decided during the
course of radiotherapy based on clinical findings or
changing anatomy: both indications for ART will be
discussed in the lecture. Unanswered questions and
caveats will be covered, e.g. how to report and interpret
final delivered doses, how to handle with volume
shrinkage in targets/organs-at-risk and how to identify
triggers to decide to adapt treatment. During the lecture,
practical tips and tricks for implementation of ART and
multimodality IGRT will be given.
SP-0207 Do we have the tools for safe application of
adaptive radiotherapy?
L.B. Hysing
1,2
, S. Thörnqvist
1,2
1
Haukeland University Hospital, Oncology and Medical
physics, Bergen, Norway
2
University of Bergen, Physics and Technology, Bergen,
Norway
Radiotherapy (RT) is traditionally administered as an
‘open loop-process’ of pre-treatment imaging, planning
and fractionated treatment delivery. But why don’t we
just image the patient at each treatment fraction, make
a plan on the fly and administer this plan to the patient
while he/she is lying on the treatment table? In the
context of adaptive radiotherapy (ART) this would be
referred to as online re-planning. ART is the process where
the original treatment plan can be modified if motivated
by feedback from previous fractions during the course of
RT (Yan et al. PMB 1997;42:123-32). Whereas feedback in
the vast majority of the clinical ART workflows in pelvic
RT are based on daily images acquired at each fraction,
the type, timing and frequency of adaptations can vary
greatly from daily online tracking and re-planning
approaches, daily plan selection or updating of the plan
once during the course of treatment (Thörnqvist S. Acta
Oncol 2016;55:943-58). As of January 2015, the online re-
planning scenario above had been applied to 1409
patients, all receiving brachytherapy for gynecological
cancer. However, for external beam therapy online re-
planning was common among in-silico simulation studies
(36% of prostate studies, 56% of gyne studies and 22% of
bladder studies as of Jan 2015), but it had not yet been
applied clinically. Identified bottle necks for clinical
application were limited in-room imaging quality (mostly
CBCT) together with manual contouring which was a pre-
requisite in 70% of the in-silico studies. For external
photon therapy, MRI is becoming an alternative to CBCT
with better soft tissue contrast thus also aiding contour
propagation based on deformable image registration.
However, for particle therapy where ART is expected to
be needed more frequently as well as for a larger fraction
of patients, in-room imaging will remain a big challenge.
For photon therapy, tools are being developed by both
research teams and vendors to allow for fast re-planning
at each treatment fraction. Such solutions should include
i) target generation ii) evaluation of the dose distribution
iii) QA of the MU calculation of beam parameters. Is such
a workflow feasible and realistic in clinical practice? Is it
safe? How and which dose should be reported and