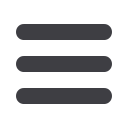

S212
ESTRO 36 2017
_______________________________________________________________________________________________
treatment options have failed. For that reason, only
sporadic cases with advanced disease and large bulky
tumours have been treated with grid therapy. Large in-
beam peak doses,
e.g.
15 Gy, have been given to the
target volume in a single treatment fraction. Although
certain sub-volumes of the target (in-between the beams)
are given low doses, significant reductions of the size of
large tumours have been demonstrated. Grid therapy has
been found to produce limited toxicity in the surrounding
sensitive tissues. The high normal-tissue tolerance to
beam grids is closely related to the so-called dose-volume
effect which has been characterized for single beams.
Experiments with beam sizes in the millimetre to
centimetre range with both proton- and photon-beams
have demonstrated that the tolerance doses for various
biological endpoints,
e.g
. different skin reactions and
nervous-system white-matter necrosis, are rising with
reduced beam sizes. Research carried out within the
American space exploration program in the 1950s showed
that the tolerance doses increased dramatically for
micrometer-wide beams. Certain endpoints,
e.g.
moist
desquamation, are never observed for sufficiently small
beam widths, even for extremely high doses. The
migration of cells from unirradiated to irradiated volumes
and an improved vascular repair if only a short segment of
a vessel is irradiated have been stated as reasons for the
improved tissue repair observed. Experiments and
preclinical radiotherapy trials with photon- and ion-beam
grids, containing beam elements of widths in the
micrometre to millimetre range, have recently been
carried out. These experiments have demonstrated the
high degree of normal-tissue tolerance to irradiation with
grids of narrow beams up to doses (hundreds of Grays)
which are much higher than those used clinically. In
ongoing radiobiological research, there are still some open
questions regarding the tumour response to spatially
modulated beams. A differential effect on the tumour
vasculature has been reported. The so-called bystander
effect has also attracted many researchers´ interests.
However, the direct effects of radiation, due to radiation
that directly hits the cell nuclei and cause DNA double-
strand breaks, are generally believed to be much more
important for the cell survival. Whether the bystander
effect significantly changes the outcome of grid therapy
has not been proven because both beneficial and
destructive bystander responses have been reported. The
x-ray beams produced by the early machines were of low
energy and highly divergent. Therefore, the beam
elements in the grid began to overlap already close to the
patient surface. The large divergence made it difficult to
produce a tissue-sparing effect at larger depths and also
made it nearly impossible to produce a uniform dose in the
target by cross-firing irradiation grids with opposing
beams. Nowadays, more parallel x-ray beams of high
energy and fluence rate are available. Thus, there is a real
possibility to exploit the normal-tissue sparing effect of
radiation grids for the treatment of more deep-seated
organs. Development in beam technology has provided
new possibilities to cross-fire radiation grids with the aim
of producing a uniform dose in the target volume.
Furthermore, beams containing charged particles,
e.g.
electrons, protons and carbon ions, have recently been
suggested for use in grid therapy. The limited range, and
the sometimes increased radiobiological effectiveness of
charged-particle beams, may be found advantageous.
Some results from the most recent research on grid
therapy will be shown in this presentation.
SP-0402 Strategies for radiosensitization with gold
nanoparticles
S. Krishnan
1
1
UT MD Anderson Cancer Center Radiation Physics,
Houston- TX, USA
Radiation therapy is a long-established component of
modern therapy for localized cancers. However, its
ultimate utility is limited by the inherent resistance of
some cancer cells to ionizing radiation. To circumvent this
problem, radiation dose escalation, targeting resistance
pathways or resistant cells with novel agents, or image-
guided tumor-targeted therapy are currently being
investigated. Emerging evidence from an explosion of
knowledge and research regarding oncologic uses of gold
nanoparticles suggests that unique solutions to each of
these problems of radiation resistance can be formulated
via the use of gold nanoparticles. Gold nanoparticles can
be used to augment the efficacy of radiation therapy via
physical dose enhancement based on an increase in
photoelectric absorption due to the high atomic number
(Z) of gold that accumulates preferentially within the
tumor due to passive extravasation of nanoparticles
through “leaky” tumor vasculature. This radiation dose
enhancement can be heightened via biological
targeting. Enhancement of radiation therapy efficacy can
also be achieved via extrinsic actuation of tumor-homing
nanoparticles to generate mild temperature hyperthermia
which enhances vascular perfusion and reduces hypoxia
initially and causes vascular disruption subsequently to
improve radioresponse. The extrinsic energy source is light
for colloidal gold nanoparticles with a large absorption
cross section that absorb and scatter light strongly at a
characteristic wavelength (their plasmon resonance) and
have a high thermal conductivity to couple this heat to the
surrounding tissue.
The interface between nanotechnology and radiation
oncology warrants continued investigation by
interdisciplinary teams of physicists, chemists, biologists,
clinicians, and engineers in industry and academia. This
talk will review the current understanding of the use of
gold nanoparticles as radiosensitizers, and outline a path
to potential clinical translation of these concepts of
radiation sensitization.
SP-0403 Potentials of Cerenkov imaging in
radiotherapy
A. Spinelli
1
1
Fondazione Centro San Raffaele, Medical Physics,
Milano, Italy
In this talk we will provide an overview of Cerenkov
radiation (CR) production mechanism, we will then show
examples of Cerenkov luminescence imaging (CLI) of small
animals and humans. The potential uses of CLI for quality
assurance (QA) and real time in vivo dosimetry during
external-beam radiation therapy (RT) will be also
presented.
The mechanism of CR production is quite unique with
respect to other and more common charged particles and
matter interaction mechanisms. In this case when a
charged particle travels through a dielectric medium, it
becomes locally polarized, with the atoms comprising the
medium behaving such as elementary dipoles. If the speed
of the particle is less than the speed of light in the
medium, symmetry of the polarization results in a
negligible field at larger distances. However, if the
particle's speed exceeds that light in this medium, the
polarization field becomes asymmetric along the particle
track producing a resultant dipole field at larger distances
from the track [1].
For a beta particle travelling in water the energy threshold
for Cerenkov emission is equal to 261 keV. This energy
threshold is relatively low and thus CLI can be applied to
image most of the beta plus and minus emitters commonly
used in nuclear medicine, and as will be described in this
talk, also to provide a novel method to monitor external-
beam RT.
CLI is becoming a well-established method for preclinical
in vivo small animal optical imaging and has been also
applied to humans for example to image a patient treated