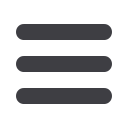

S235
ESTRO 36 2017
_______________________________________________________________________________________________
Conclusion
A fully automated planning system has been developed
that allows configuration by expert treatment planners
and oncologists. The evaluative study presented shows
high quality plans can be produced with no user input,
following the initial site-specific configuration process.
This simple process allows high-quality automated plans to
be produced for new treatment sites in an efficient
manner.
OC-0447 CyberArc: a 4π-arc optimization algorithm
for CyberKnife
V. Kearney
1
, J. Cheung
1
, T. Solberg
1
, C. McGuinness
1
1
University of California UCSF, department of radiation
oncology, San Francisco CA, USA
Purpose or Objective
To demonstrate the feasibility of 4π-arc radiotherapy
using CyberKnife for decreased treatment delivery times.
Material and Methods
A novel 4π-arc optimization algorithm (CyberArc) was
developed and evaluated in 4 prostate and 2 brain cancer
patients previously treated with CyberKnife using Iris
collimation. CyberArc was designed for continuous
radiation delivery between beam and node positions using
4π treatment geometry. During beam delivery, the
isocenter and Iris collimator diameter are allowed to
freely move within machine tolerances. For comparison
purposes, new plans were generated using the same total
number of beams and range of Iris collimation. Dose
calculation was based on the MatRad pencil beam
algorithm, modified using the machine commissioning
data to fit the CyberKnife flattening filter free beam
profiles and percent depth doses. An initial 4π library of
beam coordinates is cast over the allowed delivery
space. A constrained subplex-based optimization
algorithm then selects from an initial library of 6 node
positions for each beam coordinate using a 5mm x 5mm
fluence map resolution to obtain the first set of
beam/node/collimator configurations. A preliminary
monitor unit calculation is performed, and
beam/node/collimator positions that fall under a
threshold are discarded. A 3D traveling salesman problem
is solved using a genetic algorithm to obtain the paths
between beams (
Figure 1)
. From the second set of
beam/node/collimator
positions,
intermediate
beam/node/collimator coordinates are calculated along
the path between neighboring coordinates using cubic
interpolation. A third set of continuous intermediate
beam/node/collimator doses are calculated every 2°
along the arc path with a 2mm x 2mm fluence map
resolution. MUs are calculated for each
beam/node/collimagor position using an L-BFGS-B
optimization engine. All plans were normalized to the 70%
dose volume of the PTV for comparison.
Figure 1. The set of final beam positions and their
corresponding paths for prostate patient 3.
Results
Among the six patients analyzed, the average difference
in PTV min dose, max dose, and V95 was 2.47% ± 2.13%,
4.11% ± 2.62%, and 1.63% ± 3.01% respectively. The
average conformity index (CI) was 1.09 ± 0.07 for the brain
patients and 1.12 ± 0.09 for the prostate patients.
Figure
2
shows the plan comparison DVHs for a prostate and brain
patient. On average CyberArc decreased treatment times
by 1.76x ± 0.23x for the prostates cases and 1.62x ± 0.13x
for brain patients, not taking into consideration the gantry
speed limitations. Staying within the tolerance of the
machine speed specifications, the average time decrease
was 1.56x ± 0.19x for prostate patients and 1.39x ± 0.11x
for brain patients.
Figure 2. DVH comparison between the original CyberKnife
plan (solid line) and the corresponding CyberArc plan
(dashed line).
Conclusion
CyberArc is able to deliver plans that are dosimetrically
comparable to their CyberKnife counterparts, while
reducing treatment times considerably.
OC-0448 Near real-time automated dose restoration in
IMPT to compensate for daily tissue density variations
T. Jagt
1
, S. Breedveld
1
, S. Van de Water
1
, B. Heijmen
1
,
M. Hoogeman
1
1
Erasmus MC Cancer Institute, Radiation Oncology,
Rotterdam, The Netherlands
Purpose or Objective
Intensity-modulated proton therapy (IMPT) allows for very
localized dose deposition, but is also highly sensitive to
daily variations in tissue density along the pencil beam
paths, induced for example by variations in organ filling.
This potentially results in severe deviations between the
planned and delivered dose. To manage this, we
developed a fast dose restoration method that adapts the
treatment plan in near real-time.
Material and Methods
The dose restoration method consists of two steps: (1)
restoration of the geometrical spot positions (Bragg peaks)
by adapting the energy of each pencil beam to the new
water equivalent path length (Figure 1), and (2) re-
optimization of pencil beam weights by minimizing the
dosimetric difference with the planned dose distribution,
using a fast and exact quadratic solver.
Figure 1
Restoring spot positions. Left: The intended spot
positions. Middle: An air cavity causes a displacement and
a change in spot shape (not depicted). Right: The energy
of the pencil beam has been adapted to restore the spot
position.
The method was evaluated on 10 prostate cancer patients,
using 8-10 repeat CT scans; 1 for planning and 7-9 for
restoration. The scans were aligned based on intra-
prostatic markers. Prostate, lymph nodes and seminal
vesicles were delineated as target structures. Dose was
prescribed according to a simultaneously integrated boost
scheme assigning 74 Gy to the high-dose planning target
volume (PTV) (prostate + 4 mm) and 55 Gy to the low-dose
PTV (lymph nodes and seminal vesicles + 7 mm).
Results
While substantial dose deviations were observed in the
repeat CT scans without restoration, clinically acceptable
dose distributions were obtained after restoration (Figure