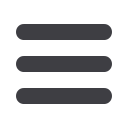

S27
ESTRO 36 2017
_______________________________________________________________________________________________
Symposium: Response adapted treatment
SP-0057 Mechanisms and biomarkers of tumour
response heterogeneity
S. Chopra
1
1
Advanced Centre for Treatment- Research and
Education in Cancer- Mumbai, Radiation Oncology,
Mumbai, India
Heterogeneity of response to therapeutic radiation is a
well-known phenomenon and is attributed to differential
evolution of tumour subpopulations that may harbor
resistant clones. Aberrant vasculature during early tumour
growth results in heterogenous microenvironment.
Tumour hypoxia and resultant acidic microenvironment
therefore becomes an early event triggering various
downstream pathways that support development and
sustainence of aggressive tumour phenotype. Hypoxic
environment is known to allow clonal evolution of stem
cell phenotype through expression of stem cell genes like
SOX-2/OCT-4/NANOG and increase in reactive oxygen
species which may in turn lead to increased DNA damage
repair and reduced cell kill after radiation, epithelial to
mesenchymal transformation and distant metastasis.
Recent research suggests that cancer stem cells may not
be in a fixed but in a dynamic state of cellular plasticity
that is dependent on the microenvironment stimulus.
Specific niching patterns for cells with stem cell
phenotype have been identified for certain tumour types.
As microenvironment may play a crucial role in nurturing
and sustaining aggressive cellular phenotypes, there may
be considerable merit in imaging and targeting
microenviornmental niches with radiation.The role of the
above possible mechanisms in radiation resistance and
biomarkers that may be linked to aggressive cellular
phenotype and tumour milieu will be discussed with
specific examples from solid tumours that are treated with
radiation.
SP-0058 Current status and future perspective of
response adaptation
D. Zips
1
1
University Hospital Tübingen Eberhard Karls University
Tübingen, Tübingen, Germany
In my presentation I will introduce the rationale of the
biological concept of delta imaging for individualized
patient management. I will discuss supporting findings and
preclinical proof-of-concept using PET/ fMRI and I will
review current clinical evidence with examples in HN,
rectal, prostate cancer.
SP-0059 Response optimised treatment planning and
guidance
B. Vanderstraeten
1
1
University Hospital Ghent, Radiotherapy - RTP, Gent,
Belgium
Biological imaging modalities like PET or fMRI aim to
unravel tumor heterogeneity, e.g. by identifying the most
radiation resistant parts of a tumor. As the total dose that
can be delivered is limited by normal tissue toxicity,
biological image-guided radiotherapy opts for dose
modification based on pre-treatment imaging or per-
treatment response assessment. The dichotomous nature
of target contouring, where voxels are either “in” or “out”
and hence intended to receive a homogeneous or no dose,
is not in agreement with reality. Dose painting by numbers
has been suggested to translate treatment response to
dose modification by means of a voxel-based dose
prescription.
Despite the present uncertainties about the applicability
of dose painting and the need for accurate biological
models, physicists and technologists should be prepared
for the challenges that per-treatment dose modification
poses to treatment planning and delivery systems. This
lecture will focus on the realization of individual dose
modification in treatment planning, including different
treatment modalities and techniques, treatment planning
system requirements, the feasibility of dose painting in
adaptive treatment schedules and automated planning.
Practical examples for head and neck and prostate cancer
will be shown.
The higher the intentional inhomogeneity of the dose
distribution, the higher the risk of getting things wrong
during treatment delivery because of set-up errors or
changes in patient anatomy. Apart from treatment
adaptation, it is important to minimize the uncertainties
in delivery and account for residual uncertainties in
planning. Using statistical models to predict tumor
presence on a voxel level, the robustness against
geometric errors can be improved. Because of the existing
technical challenges, extensive collaboration between
radiologists, radiation biologists, radiation oncologists and
physicists is needed.
Proffered Papers: Dosimetry and detector development
for particle therapy
OC-0060 Reference dosimetry of proton pencil beams
based on dose-area product
C. Gomà
1
, S. Safai
2
, S. Vörös
3
1
University Hospitals Leuven, Department of Radiation
Oncology, Leuven, Belgium
2
Paul Scherrer Institute, Center for Proton Therapy,
Villigen PSI, Switzerland
3
Federal Institute of Metrology METAS, Ionising
Radiation, Bern-Wabern, Switzerland
Purpose or Objective
To study the feasibility of a novel approach to the
reference dosimetry of proton pencil beams based on
dose-area product (DAP
w
)—the integral of the absorbed
dose to water (D
w
) over the plane perpendicular to the
beam direction. The DAP
w
of a proton pencil beam is a
quantity needed for beam modeling in most TPS.
Currently, it is calculated indirectly through the
determination of D
w
in a broad composite field together
with the reciprocity theorem. This work investigates the
direct determination of DAP
w
with reference dosimetry.
Material and Methods
The reciprocity theorem establishes an analytical
relationship between (i) the DAP
w
of a single proton pencil
beam and (ii) the D
w
at the center of a broad composite
field generated by the superposition of proton pencil
beams regularly-spaced at a distance of δx and δy (DAP
w
=
D
w
δx δy). The feasibility of reference dosimetry based on
DAP
w
(DAP
w
= M
Q
· N
DAP,w
· k
Q
) was therefore assessed by
comparison with the standard and well-established
reference dosimetry in a composite 10x10 cm
2
field based
on D
w
(D
w
= M
Q
· N
D,w
· k
Q
). First, we calibrated a PTW Bragg
Peak chamber (BPC) and a PTW Markus chamber in a PSDL
60
Co beam, in terms of DAP
w
and D
w
respectively. Second,
we calculated the beam quality correction factor (k
Q
) of
the two ionization chambers using Monte Carlo simulation.
Finally, we compared (i) the direct determination of DAP
w
of a single pencil beam using the BPC, and (ii) the indirect
determination of DAP
w
(DAP
w
= D
w
δx δy) using the Markus
chamber to determine D
w
at the center of a broad
composite field. The two approaches were compared for
proton energies ranging from 70 to 230 MeV.
Results
The BPC was successfully calibrated in terms of DAP
w
in a
60
Co beam. The uncertainty of the calibration coefficient
was estimated to be 0.2% larger than in the standard case,
due to the uncertainty in the BPC sensitive area. Figure 1
shows that direct and indirect determination of DAP
w
were