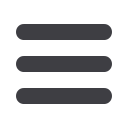

S28
ESTRO 36 2017
_______________________________________________________________________________________________
found to agree within one standard uncertainty or better,
for all proton energies.
Figure 1:
Beam monitor chamber calibration curve in
terms of DAP
w
(at z
ref
= 2 cm) per MU, as a function of
nominal proton energy, obtained with the Bragg Peak
chamber (direct) and Markus chamber (indirect). The
uncertainty bars correspond to one standard uncertainty.
Conclusion
This work proves the feasibility of the reference dosimetry
of proton pencil beams based on DAP
w
, as it agrees with
the standard and well-established approach based on D
w
within one standard uncertainty. Its main advantage is
that it is not affected by the uncertainty in beam position,
which results in an uncertainty in δx and δy. Its main
drawback is the slightly larger uncertainty of the
ionization chamber calibration coefficient. This drawback,
however, could potentially pay off in the dosimetry of
small photon fields, where perturbation factors of small
detectors might result in a larger source of uncertainty.
OC-0061 Development of a 3D plastic Scintillator
detector for a fast verification of ocular proton beam
H. Ziri
1
, D. Robertson
1
, S. Beddar
1
1
MD Anderson Cancer Center, Department of radiation
physics, Houston, USA
Purpose or Objective
Scintillator detectors have been recently used for beam
verification in radiation dosimetry. However, when
irradiated with charged particles, scintillators undergo
an ionization quenching effect that causes a decrease of
the scintillation light emission with increasing linear
energy transfer (LET). The goal of this project is to
develop a tool for ocular proton beam quality assurance
(QA) using a solid plastic scintillator without the need for
quenching correction.
Material and Methods
Figure:
Landmarks identification for range and SOBP
width measurements
The measurements were done at the Proton Therapy
Center-Houston (PTC-H). The detector system consists of
a 5x5x5 cm
3
cube of a plastic scintillator, EJ-260, that
converts the incident proton beam into visible light and a
telecentric lens coupled to a CCD camera to image the
emitted light distribution. Landmarks were determined
directly on the quenched light-depth distribution to
determine the range and spread-out Bragg peak (SOBP)
width of the beam. A common behavior of having an
inflexion point at the distal edge was noticed in the
measured scintillation light profiles. The range was
determined as the corresponding depth of that point. To
identify the inflexion point, a linear function was fitted to
the distal edge. Then, to determine the SOBP width, the
assumption that quenching is negligible at the beginning
of the SOBP was considered. A Gaussian fit was used to
smooth the curve and get better estimation of the starting
point of the SOBP. An empirical analysis showed that the
use of the 99% level of the proximal part (p99) of the
normalized light curve, as the starting point of the SOBP
seemed more appropriate to get accurate SOBP width
measurement. The measured SOBP width corresponds
then, to the distance between that identified point and
the measured range.
Figure:
Landmarks identification for range and SOBP
width measurements
Results
The validity and the accuracy of this method was
evaluated by comparison to ionization chamber
measurements. The measured ranges were in good
agreement with the ionization chamber measurements.
The mean difference was within 0.05 cm and the standard
deviation was within 2%. The measured SOBP widths, for a
nominal range of 3.5 cm, were compared to the ionization
chamber measured SOBP widths. The mean difference was
within 0.06 cm and the standard deviation was less than
4%. SOBP width measurements were also in a good
agreement with ionization chamber measurements.
Conclusion
Even though quenching decreases the emitted light with
the increase of LET with depth, landmarks can still be
identified for fast beam verification without the need for
quenching correction. It has been shown that the
developed approach works for different beam energies
with a sufficient accuracy and reproducibility for clinical
use. The accuracy of this approach is within 0.06 cm for
range and SOBP width measurement for the ocular proton
beam.
OC-0062 Correcting for linear energy transfer
dependent quenching in 3D dosimetry of proton
therapy
E.M. Høye
1
, M. Sadel
2
, L.P. Muren
1
, J.B.B. Petersen
1
, P.
Skyt
1
, L.P. Kaplan
2
, J. Swakon
3
, L. Malinowski
3
, G.
Mierzwińska
3
, M. Rydygier
3
, P. Balling
2
1
Aarhus University Hospital, Medical Physics, Aarhus C,
Denmark
2
Aarhus University, Department of Physics and
Astronomy, Aarhus, Denmark
3
Polish Academy of Sciences, Institute of Nuclear Physics,
Krakow, Poland
Purpose or Objective
Three dimensional (3D) dosimetry allows for detailed
measurements of dose distributions in photon-based
radiotherapy, and has potential to become a useful tool
for verification of proton therapy (PT). However, linear
energy transfer (LET) dependent quenching of the signal
in the Bragg peak results in an under-response of the
dosimeter. In this study we investigate whether the LET