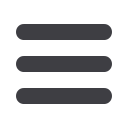

S401
ESTRO 36 2017
_______________________________________________________________________________________________
Conclusion
The results of our study show that 3D printing technology
can be used to fabricate patient-specific, large scale
phantoms that could be used for a variety of research,
dosimetric,
and
quality
assurance
purposes.
PO-0766 The effect of air gaps on Magic Plate (MP512)
for small field dosimetry
K. Utitsarn
1
, N. Stansook
1
, Z. Alrowaiili
1
, M. Carolan
2
, M.
Petasecca
1
, M. Lerch
1
, A. Rosenfeld
1
1
University of Wollongong, Center for Medical Radiation
Physics, Wollongong, Australia
2
Wollongong Hospital, Illawara Cancer Care Centre,
Wollongong, Australia
Purpose or Objective
We evaluate the impact of an air gap on the MP512
irradiation response at depth in a phantom and optimize
this gap for accurate small field dosimetry in clinical
photon and electron beams.
Material and Methods
MP512 is a 2 dimensional silicon monolithic detector
manufactured on a p-type substrate. The array consists of
512 pixels with detector size 0.5x0.5 mm
2
and pixel pitch
2 mm. The overall area of the active part of the detector
is 52x52 mm
2
. The output factor (OF) and the percentage
depth dose (PDD) were measured with MP512 in 6MV and
10MV photon beams. The OF was measured at a depth of
10 cm in a solid water phantom for square field sizes
ranging from 0.5 to 10 cm
2
. The PDD was measured for
field sizes 2x2cm
2
, 5x5cm
2
and 10x10cm
2
by scanning the
MP512 from the depth of 0.5 cm to 10 cm. Both the OF and
PDD were measured at all field sizes with an air gap
immediately above the detector of 0.5, 1.0, 1.2, 2.0 and
2.6 mm respectively. The PDD for 6, 12 and 20 MeV
electron beams with a standard applicator providing 10x10
cm
2
field size, were measured using an air gap of 0.5mm
and 2.6mm.
Results
The OF measured by the MP512 reduces with increasing air
gap above the detector. The impact of the air gap is
largest for the small fields of 0.5x0.5 and 1x1 cm
2
while
negligible for field sizes larger than 4x4 cm
2
. The OF
measured by the MP512 detector with an air gap of 0.5 and
1.2 mm show a good agreement with OF measured using
EBT3 film and MO
Skin
for 6 and 10 MV, respectively.
Similar results were observed for the PDD measurements
in field sizes of 5x5 and 10x10 cm
2
. The PDD for a 2x2 cm
2
was within ±3% of the EBT3 for both photon energies. The
PDD measured with MP512 is within ±1.6% and ±1.5% of
that measured using a Markus ionization chamber (IC) for
6 and 10 MV fields respectively. The PDD measured by
electron beams demonstrated no significant effect with
increasing air gap above the MP512 for all energies. The
results for both 0.5mm and 2.6mm gap are within ±3% of
similar measurements made using the Markus IC.
Conclusion
The MP512 response with different air gaps immediately
above the detector in solid water phantom have been
investigated in clinical photon and electron fields. The
results confirm that the MP512 monolithic diode array is
suitable for QA of small fields in a phantom. The study
shows that the air gap size has a significant effect on small
field photon dosimetry performance of the MP512
consistent with a loss of electronic equilibrium. The small
air gap of 0.5 mm and 1.2 mm is the best air gap for small
field dosimetry in 6 and 10 MV photon beams respectively.
The effect of air gap on electron beam dosimetry using the
MP512 was demonstrated to be not significant due to the
electronic equilibrium conditions always being fully
established.
PO-0767 Revisiting EPID design for modern
radiotherapy requirements
P. Vial
1,2
, S. Blake
2,3
, Z. Cheng
2,3
, S. Deshpande
1,4
, S.
Atakaramians
5
, M. Lu
6
, S. Meikle
7
, P. Greer
8,9
, Z. Kuncic
2
1
Liverpool and Macarthur Cancer Therapy Centres and
Ingham Institute, Department of Medical Physics,
Liverpool BC, Australia
2
University of Sydney, Institute of Medical Physics-
School of Physics, Sydney, Australia
3
Ingham Institute, Medical Physics, Liverpool, Australia
4
University of Wollongong, Centre for Medical Radiation
Physics, Wollongong, Australia
5
University of Sydney, Institute of Photonics and Optical
Science- School of Physics, Sydney, Australia
6
Perkin Elmer, Medical Imaging, Santa Clara, USA
7
University of Sydney, Faculty of Health Sciences & Brain
and Mind Centre, Sydney, Australia
8
University of Newcastle, School of Mathematical and
Physicsal Sciences, Newcastle, Australia
9
Calvary Mater Newcastle Hospital, Radiation Oncology,
Newcastle, Australia
Purpose or Objective
New methods of treatment verification that are in keeping
with advances in radiotherapy technology are desirable.
The availability of kilovoltage in-room imaging for
example has led to a general trend away from the poorer
contrast megavoltage (MV) imaging for patient-set-up.
The widespread use of intensity-modulated radiotherapy
(IMRT) also reduces the utility of treatment beams as a
source of imaging for treatment verification. At the same
time there has been a steady increase in the use of
electronic portal imaging devices (EPIDs) for dose
verification. There is however emerging evidence of new
roles for MV imaging in real-time target tracking. In this
work we address the issue of EPID detector specifications
in light of changing clinical requirements. We present a
general overview of the detector development work our
group has undertaken to design an EPID that better
supports applications relevant to current and future
clinical practice.
Material and Methods
Prototype EPID technologies developed by our group
include: a direct detector EPID where the metal/phosphor