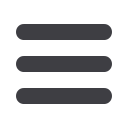

S404
ESTRO 36 2017
_______________________________________________________________________________________________
Campus Pius Hospital Carl von Ossietzky University,
Oldenburg, Germany
2
Prof em. Medical Physics and Biophysics, Georg August
University, Göttingen, Germany
Purpose or Objective
The development of therapeutic devices combining
clinical linear accelerators and MRI scanners for MR guided
radiotherapy leads to new challenges in the clinical
dosimetry since the trajectories of the secondary
electrons are influenced by the Lorentz force. In this
study, the lateral dose response functions of a clinical air-
filled ionization chamber in the presence of a magnetic
field were examined depending on beam quality and
magnetic field following the approach of a convolution
model (Looe
et al
2015, Harder
et al
2014).
Material and Methods
In the convolution model, the 1D lateral dose response
function
K
(
x-ξ
) is defined as the convolution kernel
transforming the true dose profile
D
(
ξ
) into the disturbed
signal profile
M
(
x
) measured with a detector. For an air-
filled ionization chamber, type T31021 (PTW Freiburg,
Germany), the lateral dose response functions were
determined by Monte-Carlo simulation using 0.25 mm wide
60
Co and 6 MV slit beams. The chamber was modelled
according to manufacturer’s detailed specification and
placed at 5 cm water depth in three different
orientations, i.e. axial, lateral and longitudinal. For each
chamber orientation, a magnetic field oriented
perpendicular to the beam axis was applied. Simulations
were performed for magnetic fields of 0, 0.5, 1 and 1.5 T
using the EGSnrc package and the
egs_chamber
code.
To verify the simulation results, the lateral dose response
functions without magnetic field were compared against
measurements with a 5 mm wide collimated 6 MV photon
slit beam using tertiary lead blocks following the approach
of Poppinga
et al
2015.
Results
Fig. 1 shows good agreement between the simulated and
measured dose response functions
K
(
x-ξ
) of the
investigated ionization chamber in the three investigated
orientations. The structures of the measured functions are
not as evident as those of the simulated functions possibly
due different scanning step widths used in the experiment
and the calculation.
Fig. 2 shows the lateral dose response function
K
(
x-ξ
) with
and without magnetic field obtained exemplary for the
detector in lateral orientation. The distortion of the dose
response function
K
(
x-ξ
) corresponds to the change in the
trajectory lengths of the secondary electrons in the air of
the ionization chamber due to the Lorentz force, as
compared to the trajectories in a small sample of water.
Fig. 1. Area-normalized simulated and measured dose
response functions
K
(
x
-
ξ
)
Fig. 2. Area-normalized dose response functions
K
(
x
-
ξ
) for
the T31021 in lateral orientation for magnetic fields of 0,
0.5,1 and 1.5 T
Conclusion
The distortions of the lateral dose response function
K
(
x-
ξ
) will alter the measured signal profile
M
(
x
) of a detector
in magnetic field, as demonstrated in this study. The
variety of the possible combinations of detector
orientation and magnetic field direction and the strong
dependence of the distortion on the magnetic field
strength require careful consideration whenever a non-
water equivalent detector is used in magnetic field.
PO-0772 Patient-specific realtime error detection for
VMAT based on transmission detector measurements
M. Pasler
1
, K. Michel
2
, L. Marrazzo
3
, M. Obenland
4
, S.
Pallotta
5
, H. Wirtz
4
, J. Lutterbach
6
1
Lake Constance Radiation Oncology Center, Department
for Medical Physics, Friedrichshafen, Germany
2
Lake Constance Radiation Oncology Center- Martin-
Luther-Universität Halle-Wittenberg, Department for
Medical Physics- Naturwissenschaftliche Fakultät II,
Friedrichshafen, Germany
3
AOU Careggi, Medical Physics Unit, Florence, Italy
4
Lake Constance Radiation Oncology Center, Department
for Medical Physics, Singen, Germany
5
University of Florence- AOU Careggi, Medical Physics
Unit- Department of Biomedical- Experimental and
Clinical Sciences, Florence, Italy
6
Lake Constance Radiation Oncology Center,
Radiooncology, Singen, Germany
Purpose or Objective
To investigate a new transmission detector for online dose
verification. Error detection ability was examined and the
correlation between the changes in detector output signal
with γ passing rate and DVH variations was evaluated.
Material and Methods
The integral quality monitor detector (IQM, iRT Systems
GmbH, Germany) consists of a single large area ionization
chamber which is positioned between the treatment head
and the patient. The ionization chamber has a gradient
along the direction of MLC motion and is thus spatially
sensitive. The detector provides an output for each single
control point (segment-by-segment) and a cumulative
output which is compared with a calculated value.
Signal stability and error detection sensitivity were
investigated. Ten types of errors were induced in clinical
VMAT plans for three treatment sites: head and neck (HN),
prostate (PC) and breast cancer (MC). Treatment plans
were generated with Pinnacle (V.14) for an Elekta synergy
linac (MLCi2). Geometric errors included shifts of one or
both leaf banks for all control points toward (i) and away
(ii) from the central axis of the beam and unidirectional
shifts of both leaf banks (iii) by 1 and 2mm, respectively.
Dosimetric errors were induced by increasing the number
of MUs by 2% and 5%.
Deviations in dose distributions between the original and
error-induced plans were compared in terms of IQM signal
deviation, 2D γ passing rate (2%/2mm and3%/3mm) and
DVH metrics (D
mean
, D
2%
and D
98%
for PTV and OARs).
Results
For segment-by-segment evaluation, calculated and
measured IQM signal differed by 4.7%±5.5%, -2.6%±4.6%
and 4.19%±6.56% for MC, PC und HN plans, respectively.
Concerning the cumulative evaluation, the deviation was
-1.4±0.25%, -6.0±0.3% und -1.47%±0.97%, respectively.
Signal stability for ten successive measurements was
within 0.5% and 2% for the cumulative and the segment-
by-segment analysis.
The IQM system is highly sensitive in detecting geometric
errors down to 1mm MLC bank displacement and
dosimetric errors of 2% if a measured signal is used as
reference. Table 1 reports IQM signal deviations for a
range of introduced errors.