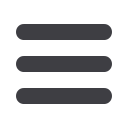

S406
ESTRO 36 2017
_______________________________________________________________________________________________
rates. Irradiations were performed with a
60
Co PICKER unit
in a secondary standard calibration laboratory. The
samples were divided into groups of two and each group
was placed at a different distance (56.65 - 427 cm) from
the
60
Co source at a 5cm depth within a water phantom.
Irradiation times varied in order to deliver the same dose
of 1 Gy at the center of all cuvettes with dose rates in the
range of 0.018 – 1.0 Gy/min. For the high dose rate study,
a similar methodology was employed. Four couples of
PRESAGE cuvettes were placed within a slab in a solid
water phantom and irradiated at different dose-rates by
varying the dose delivery rate of an ELEKTA Versa HD FFF
linac from 2.5 up to 19 Gy/min. Dose delivery of 1 Gy for
all dose rates was verified by ion chamber measurements.
Irradiation induced optical density (OD) change was
measured from pre- and post-irradiation scans with a
digital spectrophotometer operated at 633 nm. Mean OD
change for each group was normalized to the value for the
highest dose rate in each study.
Results
Results presented in figure 1 show a trend of increasing
PRESAGE dose sensitivity with decreasing dose rate with
the over-response reaching up to 16% at 0.018 Gy/min.
Although in a first approach such low dose rates could be
considered extremely low in external radiotherapy, recent
studies have shown that in advanced radiotherapy
techniques (e.g. VMAT) dose rate varies drastically across
dose distributions delivered and a considerable
contribution of the delivered dose could come from very
low dose rates (0.01 - 0.1Gy/min). Regarding the high dose
rate study, all responses agree within experimental
uncertainties, indicating that PRESAGE sensitivity is not
significantly affected.
Figure 1: Dose rate dependence of PRESAGE response for
both studies included in this work. Error bars correspond
to 1 standard deviation of all experimental uncertainties
involved.
Conclusion
Results of this study indicate a significant over-response
of this PRESAGE formulation in very low dose rates that
should be considered when they are used in applications
involving wide range of dose delivery rates.
Acknowledgement: This work was financially supported by
the State Scholarships Foundation of Greece through the
program ‘Research Projects for Excellence IKY/SIEMENS’.
PO-0775 Contributions to detector response in
arbitrary photon fields
S. Wegener
1
, O.A. Sauer
1
1
University Hospital, Radiation Oncology, Würzburg,
Germany
Purpose or Objective
Due to their small active volumes, diodes are often the
detectors of choice for many commissioning tasks
including the measurement of output factors, especially in
small fields. However, high-atomic number material in the
chip, detector shielding or other components and a finite
active volume size have been found to alter the signal
compared to the dose ratios measured in water in the
absence of such a detector. As a consequence, correction
factors need to be applied to correct the obtained signals.
Using three experimental setups (fig. 1), the different
contributions to the detector signals were separated and
analyzed: the response to scatter, the primary beam and
the combination of both.
Material and Methods
Signal ratios were obtained for three different
experimental setups (fig. 1): First, the standard open field
geometry. Secondly, fields in which the central part of the
beam was blocked out by a 4 mm aluminum pole and the
detector was positioned in the dose minimum
below. Finally, the detector in air instead of water with
a PMMA cap fitted on top. A range of typically used
detectors were analyzed, namely a microDiamond, a
PinPoint ionization chamber, an EDGE diode, as well as
three shielded and three unshielded diode detectors. EBT3
Gafchromic film served as reference. Measurements were
carried out on a PRIMUS linac at a photon beam quality of
6 MV with field sizes between 0.8 and 10 cm.
Responses in the blocked field and the PMMA setup were
combined to calculate the response in the open square
fields. The results were interpolated to a general matrix
from which responses in any field could be calculated.
Examples of such fields were measured for comparison.
Results
A higher detector overresponse and increasing detector to
detector differences were observed when the primary
beam was blocked out, whereas almost identical response
was seen for all detectors in the primary beam. A
combination of the responses in those two setups in a
detector-dependent ratio reproduced the values obtained
in the open field geometry with less than 1% deviation for
all detectors studied and all quadratic field sizes. For
rectangular and offset fields the agreement is still almost
within 1 %. Only when the detector was close to the field
edge larger deviations occurred (fig. 2).
Conclusion
Detector responses in open fields could be calculated from
the response to scatter and in the primary beam with 1%
agreement in all studied square fields and for all studied
detectors. The calculation was extended to rectangular
and non-symmetric fields yielding results in agreement
with the measurements for a wide range of fields. This
method suggests a way to calculate correction factors for
arbitrary fields.