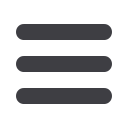

S757
ESTRO 36 2017
_______________________________________________________________________________________________
Purpose or Objective
Anthropomorphic phantoms are used in a variety of ways
in radiation therapy for both research and quality
assurance purposes. Most anthropomorphic phantoms are
of generalized patients, but 3D printing technology can be
used to fabricate patient-realistic phantoms for special QA
and verification procedures. Most 3D printers, however,
can only print in one or two materials at a time, so true
patient heterogeneity is limited. In this study, we
examined two different patient specific, 3D printed
phantoms created based on the same patient to determine
the accuracy of single and multi-material phantoms.
Material and Methods
The phantoms used in this study were designed from the
clinical CT data for a post-mastectomy patient treated at
our institution. The CT data was trimmed to remove the
patient’s head and arms to preserve anonymity and
simplify printing. Phantom 1 was designed by converting
the trimmed CT data into a 3D model with a CT threshold
of >-500 Hounsfield units (HU). This model was sliced into
2.5-cm-thick sagittal slices and printed one slice at a time.
All slices were printed with polylactic acid (PLA)
representing all body tissues, but with air cavities and
lower density regions like the lungs left open. Sagittal
slices were chosen for their superior fit with each other,
and minimal material warping relative to axial slices.
Phantom 2 was designed by converting the CT data into
three separate 3D models with a CT threshold of <-147 HU
for air cavities, -147 to 320 HU for soft tissue, and >320
HU for bone. The models were sliced into 1-cm-thick axial
slices, and printed. The slices were printed from the soft
tissue model using a custom formulated high impact
polystyrene (HIPS) with the air and bone models left open.
After printing, the open bone model sections were filled
with a liquid resin polymer with an equivalent density to
bone.
The phantoms were evaluated for their materials and
overall accuracy to the original patient CT. Blocks of PLA,
HIPS, and the bone resin material were all imaged to
determine their average HU. The phantoms were also each
imaged and registered with each other and the original
patient CT to determine the consistency and accuracy of
each phantom.
Results
The materials used and their properties are summarized
in Table 1. Phantom 1 was fabricated from PLA, which
isn’t particularly tissue equivalent, but did print relatively
consistently. The bone resin and HIPS of phantom 2 more
accurately reflect tissue heterogeneity, but have more
variations in their printed consistency.
Figure 1 shows registered images of the original patient
image (a), phantom 1 (b), and phantom 2 (c). Tissue
density was more accurate in phantom 2, despite some
small holes not being filled with bone resin.
Conclusion
Two phantoms were created, one with a single material,
and a second with two materials (tissue and bone). These
two phantoms provide an ability to more closely simulate
the patient and provide a means to more accurately
measure dose delivered in a patient surrogate.
EP-1436 A newly designed water-equivalent bolus
technique enables BNCT application to skin tumor.
K. Hirose
1
, K. Arai
1
, T. Motoyanagi
1
, T. Harada
1
, R.
Shimokomaki
1
, T. Kato
1
, Y. Takai
1
1
Southern TOHOKU BNCT Research Center, Radiation
Oncology, Koriyama, Japan
Purpose or Objective
The accelerator-based boron neutron capture therapy (AB-
BNCT) system was developed in order to enable the
installation of safe hospital BNCT. An important feature of
AB-BNCT system is its capability of delivering great doses
to deep-seated tumors under condition in which a
beryllium target and neutron-beam-sharping assembly are
adjusted for production of epithermal neutron that is
applicable
for
more
types
of
tumor
localization.Conversely, AB-BNCT is less suitable for
superficial cancers, such as malignant melanoma. In this
study, we developed a newly water-equivalent bolus
technique that has no production of prompt gamma ray
and no influence on complicating dose calculation, and we
evaluated the effect of this technique on treatment
quality for a case of malignant melanoma patient.
Material and Methods
A water-equivalent bolus was prepared as follows.
Urethane foam was cut down into the size of 3-cm larger
than the superficial lesion, infiltrated with distilled water
with deaeration, and covered with a thin film. The
simulated patient was played by a healthy man and
simulated condition was originated from a malignant
melanoma patient with the lesion of 3-cm diameter
localized in a sole of right foot. The superficial lesion was
bordered by a catheter and covered with a water-
equivalent bolus. Using treatment planning system SERA,
the tumor is depicted as a region surrounded by the
catheter with 5-mm thickness, and also skin is depicted as
the other region except for tumor with 3-mm thickness
from body surface. A water-equivalent bolus was
delineated as water. This was placed into air in calculation
in condition with no bolus. For comparison with bolus-like
effect of a covered collimator, the outline of an imaginary
collimator cover was set as a mass of polycarbonate or a