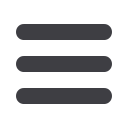

S768
ESTRO 36 2017
_______________________________________________________________________________________________
the OBI from Varian’s TrueBeam system. Simulations are
to be validated using in phantom OSL measurements.
Material and Methods
For both kV-CBCT systems, the kV irradiation head
geometry was implemented in the MC simulation code
Penelope. As a first step, the resulting photon
distributions were expressed as Virtual Source Models
(VSM) for every standard irradiation condition (kVp,
filtration, collimation); it was then validated and adjusted
using in water-phantom measurements performed wit a
calibrated Farmer-type ionization chamber.
In a second step, the validated VSMs were used to simulate
the dose delivered by both the XVI and OBI systems in
anthropomorphic phantoms, using standard clinical
imaging protocols. Simulated dose-to-organs were then
confronted to dose measurements performed using OSL
inserted into the same phantoms, following a dosimetric
protocol for OSLs previously established [1].
In addition, VSM results were confronted to their direct MC
counterparts in order to evaluate the benefit of using such
technique.
Results
The current study highlights the possibility to reproduce
OSL dose-to-organ measurements using VSM-driven Monte
Carlo simulation with an overall agreement better than 20
%. In addition, the use of VSM in the MC simulation enables
to speed-up the calculation time by a factor better than
two (for the same statistical uncertainty) compared to
direct MC simulation. Nevertheless, if direct and VSM
calculations are in agreement inside the irradiation field,
outside, VSM results tend to be significantly lower (10-
30%).
Conclusion
The use of a VSM was demonstrated to simplify and fasten
MC simulations for personalized kV-CBCT MC dose
estimation. In addition, OSLs enable to perform the low
dose measurement in the kV range needed for in phantom
X-ray imaging equipment dose QA.
This study is to be completed in the near future by the
addition of other standard X-ray imaging equipment
dedicated to IGRT.
EP-1456 In-vivo dosimetry using Dosimetry Check: 5-
year experience on 345 prostate cancer patients
W.H. Nailon
1
, D. Welsh
1
, K. MacDonald
1
, D. Burns
2
, J.
Forsyth
2
, G. Cooke
1
, F. Cutanda
1
, D.B. McLaren
3
, J.
Puxeu-Vaque
1
, T. Kehoe
1
, S. Andiappa
1
1
Edinburgh Cancer Centre Western General Hospital,
Department of Oncology Physics, Edinburgh, United
Kingdom
2
Edinburgh Cancer Centre Western General Hospital,
Department of Radiography, Edinburgh, United Kingdom
3
Edinburgh Cancer Centre Western General Hospital,
Department of Clinical Oncology, Edinburgh, United
Kingdom
Purpose or Objective
It is recommended that all radiotherapy centres in the
United Kingdom (UK) have a protocol for in vivo dosimetry
(IVD) and in several European countries IVD is now
mandatory. Electronic portal imaging devices (EPIDs),
which although developed primarily for the purposes of
imaging, are now widely used for IVD and consequently for
treatment quality assurance (QA). Here we present results
from 5-year clinical experience of using IVD for
verification of prostate cancer patients.
Material and Methods
Between 2011 and 2016 IVD was performed by Dosimetry
Check (DC) (Math Resolutions LLC, Columbia, MD, USA) on
345 prostate cancer patients. Treatment plans were
prepared in Eclipse (Varian Medical Systems, Inc., Palo
Alto, CA, USA) with 285 patients treated with a volumetric
modulated arc therapy (VMAT) technique and 60 patients
treated with a three-dimensional conformal radiotherapy
(3DCRT) technique. The difference between the dose
calculated by Eclipse at a reference point and the dose
measured by DC at the same reference point at time-of-
treatment was recorded. In cases where the dose
difference exceeded ±10% an alert was triggered and a full
three-dimensional gamma analysis (4%/4mm) performed
on the treatment plan. This led to either the measurement
being repeated or further positional and patient-specific
QA checks being performed.
Results
Figure 1 shows the percentage difference in point doses
calculated by Eclipse and measured by DC for the 3DCRT
and VMAT treatments monitored. The mean and standard
deviation (µ±σ) of the percentage difference in dose
obtained by DC and calculated by Eclipse was 1.23±4.61%
in VMAT and −3.62±4.00% in 3DCRT. A total of 12 plans
exceeded the ±10% alert criteria accounting for 3.5% of all
prostate cancer treatments monitored. In all of these
cases further investigation using 3D gamma analysis and
additional patient-specific QA found no reportable
treatment
errors.
Figure 1
: IVD point dose measurements on all prostate
cancer patients treated between 2011 and 2016.
Conclusion
The preliminary results of this pilot study show that EPID-
based IVD using the DC software has the potential to
detect errors and identify sub-optimal treatments. With
the addition of more data it may also be possible to
establish site-specific alert levels, which could improve
the quality of radiotherapy.
EP-1457 Introducing the fraction of penumbra dose in
the evaluation of VMAT treatment plans
A. Bäck
1
, F. Nordström
1
, M. Gustafsson
1
, J. Götstedt
2
, A.
Karlsson Hauer
1
1
Sahlgrenska University Hospit, Therapeutic Radiation
Physics, Göteborg, Sweden
2
University of Gothenburg, Radiation Physics, Göteborg,
Sweden
Purpose or Objective
The overall objective is to develop a 3D complexity metric
for VMAT treatments. The complexity scores will be
presented as a distribution in a 3D volume and correlate
to the fraction of penumbra dose. Regions lacking charged
particle equilibrium that might cause dose calculation
errors and regions sensitive to multileaf collimator (MLC)
positioning errors are located in the penumbra of the MLC
opening. The hypothesis is that an increased amount of
dose in a voxel that originates from a penumbra region will
correlate to the probability of increased difference
between planned and delivered dose in that voxel. In this
pilot study, 2D distributions are analyzed to validate the
correlation to differences between calculated and
measured dose.
Material and Methods
A C# software with dynamically linked MatLab®
(Mathworks, Natick, MA) libraries was developed. The