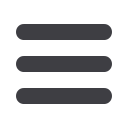

S792
ESTRO 36 2017
_______________________________________________________________________________________________
Communications Department, Alcala de Henares-Madrid,
Spain
Purpose or Objective
In this study, we present a new method for portal
dosimetry. CT images of the Electronical Portal Imaging
Device (EPID) were used as phantom images for dose
calculation. The clinical beam model and beam energy, in
the treatment planning system, were used to calculate
dose over the EPID.
Material and Methods
The method was developed for a Varian Clinac 21-EX
(Varian Medical Systems, USA), with a nominal photon
energy of 6 MV, equipped with a Varian aS1000 EPID.
Pinnacle 8.0m (Philips Medical Systems, NL) was used for
treatment planning calculations. Matlab® v2012a
(Mathworks, USA) was employed to develop code for
calculations involving backscatter and output correction
factors.
The EPID was calibrated, following the manufacturer
procedure, and then unmounted from the linear
accelerator and scanned to acquire CT images of the EPID
(Fig. 1) on an Aquilion LB (Toshiba Medical Systems,
Japan). These CT images were imported into the Pinnacle
planning system. The imported images were used as a
quality assurance phantom to calculate dose on the image
plane, which was considered as the predicted portal dose.
Two sliding-window IMRT treatment plans, a prostate and
a head and neck case, were delivered, measured and
analyzed with both with the EPID and with MatriXX (IBA
Dosimetry, Germany), as an independent measurement
method.
Matlab code was used to calculate EPID arm
backscattering and output factor corrections. Gamma
index comparison (3 %, 3 mm) was made for the EPID and
MatriXX dose planes versus the calculated dose planes with
OmniPro ImRT (IBA Dosimetry).
Figure 1. Acquired CT images of the Varian aS1000
EPID.
Results
For plans verified with EPID, Gamma index pass rate were
98.6% and 96.5% for prostate (Table 1) and head and neck
case, respectively. Dose differences (EPID vs planned)
were -0.7% and -0.4%.
For MatriXX measurements, the results are very similar:
gamma pass rate of 97.2% for prostate and 97.9% for head
and neck, and dose differences (MatriXX vs planned) of -
1.4% and -0.8%, respectively.
Field
Gamma
(3 %, 3
mm)EPID
Dose
diff EPID
(%)
Gamma (3 %,
3
mm)MatriXX
Dose
diffMatriXX
(%)
1
98.5%
-2.0
2
98.6%
-1.0
96.5%
-1.6
3
98.9%
-0.5
98.1%
-1.1
4
98.6%
-0.6
96.0%
-1.5
5
99.0%
-0.3
96.5%
-1.1
6
98.7%
-0.1
99.0%
-1.5
7
98.0%
-0.6
97.3%
-1.2
Average
98.6%
-0.7
97.2%
-1.4
Table 1. Gamma index and dose difference results for
prostate treatment.
Conclusion
The obtained results show the validity of the method
presented here. This method can be easily implemented
into clinic, as no additional modeling of the clinical beam
is necessary. The main advantage of this method is that
portal dose prediction is calculated with the same
algorithm and energy beam model used for patient
treatment planning dose distribution calculations.
EP-1497 Dosimetric effect of the Elekta Fraxion cranial
immobilization system and dose calculation accuracy
C. Ferrer
1
, C. Huertas
1
, R. Plaza
1
, A. Serrada
1
1
Hospital universitaria La Paz, Radiofísica y
Radioprotección, Madrid, Spain
Purpose or Objective
Devices external to the patient may cause an increase in
the skin dose, as well as modify the dose distribution and
hence the tumor dose. This study describes the effect on
this parameters caused by the Elekta Fraxion cranial
immobilization system. The effect of the inclusion of
Fraxion in ElektaMonaco treatment planning system (v.
5.00.00) was also checked.
Material and Methods
To study the dose attenuation a cylindrical phantom was
placed over the Elekta Fraxion with a CC13 Scanditronix-
Wellhofer ionization chamber located in the central insert
at the linac isocenter. Dose measurements were
performed for two open fields, 10x10 cm and other smaller
5x5 cm, as Fraxion is used mainly for radiosurgery
treatments. The gantry angles were the ones which cross
Fraxion (135º - 225º, 5º-10º increment, IEC gantry angles).
Calculated and measured doses are the average doses of
symmetrical angles from 180º. Reference dose without
Fraxion was the average dose at 0º, 90º, and 270º. 100 MU
were delivered at each angle. All measured doses were
compared with the ones calculated with Monaco.
To measure the skin dose and the dose distribution in the
Build-up region, several radiochromic Films EBT3 were
placed at linac CAX between the slabs of a RW3 phantom
placed over Fraxion (SSD= 90 cm) and read using FilmQA
Pro software. Films were situated at the surface, 0.5 cm,
1.5cm depth and the linac isocenter. 200 MU were
delivered for 10x10 and 5x5 open field sizes and 0º gantry
angle. Once irradiated and removed, another set of films
were placed under the phantom, in contact with Fraxion,
and at 0.5 cm and 1.5 cm from Fraxion, as well as at the
linac isocenter. Additional films were located 1 cm away
from CAX as in this section Fraxion is wider. Same field
sizes and MU at 180º were employed.
Results
Table 1 shows the comparison between measured and
calculated transmitted dose with and without Fraxion in
the calculation. Measurements show a 1% attenuation for
180º gantry angle as stated on the Fraxion manual, but this
attenuation can be as high as 5 % (5x5 open field) or 6 %
(10x10 open field) for 150º gantry angle, as with this
angle, the beam traverses the thickest part of the Fraxion.
If Fraxion is not included in the calculation, Monaco
calculation can result in a 7 % difference between
measured and calculated doses, while with Fraxion in the
calculation, the maximum difference is 1.5% (10x10,
150º).
Table 2 shows the evaluated skin dose increment caused
by Fraxion, and compares calculated and scanned values.
Fraxion increases 3.8 times the surface dose, and by 17%
at 0.5 cm depth, which can be calculated by Monaco with
a difference lower than 1% if Fraxion is included in the