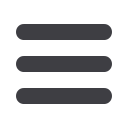

S850
ESTRO 36 2017
_______________________________________________________________________________________________
All plans for all patients reached prescription doses while
adhering to dose constraints. The average volumes of GTV-
histo, GTV-union and GTV-intersection were 7±8 ml, 9±9
ml and 3±4 ml. In Plan95
union
and Plan95
intersection
the mean
doses on GTV-histo were 95.7±1.5 Gy and 90.7±6.9 Gy,
respectively (p=0.016). Average TCP-histo values were
63±29%, 99±1% and 90±11% for Plan77, Plan95
union
and
Plan95
intersection
respectively. PLAN95
union
had significantly
higher TCP-histo values than Plan77 (p=0.016) and
Plan95
intersection
(p=0.03). There were no significant
differences in rectal and bladder NTCPs between the 3
plans.
Conclusion
IMRT dose painting for primary PCa using combined
68
Ga-
HBED-CC PSMA-PET/CT and mpMRI was technically
feasible. A dose escalation to GTV-union resulted in
significantly higher TCPs without higher NTCPs.
EP-1598 Modelisation of radiation response at various
fractionation from histopathological prostate tumors
V. Aubert
1,2
, O. Acosta
1,2
, N. Rioux-Leclercq
3
, R.
Mathieu
4
, F. Commandeur
1,2
, R. De Crevoisier
1,2,5
1
INSERM, U1099, Rennes, France
2
University Rennes 1, LTSI, Rennes, France
3
Rennes Hospital and University, Department of
Pathology, Rennes, France
4
CHU Pontchaillou, Department of Urology, Rennes,
France
5
Centre Eugène Marquis, Department of Radiotherapy,
Rennes, France
Purpose or Objective
Using simulation from histopathological cancer prostate
specimen, the objectives were to identify the total dose
corresponding to various fractionations necessary to
destroy the tumor cells (50% to 99.9%) and to assess the
impact of the Gleason score on these doses.
Material and Methods
Histopatological specimen were extracted from 7 patients
having radical prostatectomy. A senior uropathologist
manually delineated all tumor foci on the hematoxylin and
eosin-stained axial slides and assigned Gleason scores (GS)
to each individual focus. Antibodies CD31 were used as
blood vessel markers. Three slide samples per patient,
corresponding to a surface of 2000µm x1200µm, were
scanned and used within a simulation model developed in
the Netlogo software (Figure 1). The model contained the
following cells: tumor cells with a density ranging from
45% to 85%, endothelial cells with a density ranging from
0.3 to 8% and normal cells. The samples were GS:7 (3+4)
for 47.6%, GS:7 (4+3) for 28.6% and GS:8 (4+4) for 23.8%.
We used the equations of the model simulating the
radiation response of hypoxic tumors published by
Espinoza et al.
(Med Phys 2015)
. The model parameters
were adjusted to biological values from the literature:
diffusion coefficient (2.10
-9
m²/s), Vmax and Km of oxygen
consumption (15 and 2.5 mmHg), tumor cells proliferation
(1008 hours), half-life of dead cells (168 hours), α (0.15
Gy
-1
) and β (0.048 Gy
-2
) of the linear-quadratic model.
Three fractionations were tested, at 2, 2.5 and 3
Gy/fraction at 24h interval. Five simulations were
performed by slide sample. The objectives were to
identify the total dose, at each fractionation, to kill 50%
to 99.9% of the tumor cells.
Results
A total of 315 simulations were performed. Figure 2 shows
the total doses necessary to kill 50% to 99.9% of the tumor
cells, depending on the fractionation. The mean (SD)
doses (Gy) to kill 99% of the tumor cells were therefore 72
(±14), 68 (±13) and 65 (±12) for fractionations (Gy) of 2,
2.5 and 3, respectively. The mean (SD) doses (Gy) to kill
99.9% of the tumor cells were therefore 107 (±17),
101(±16) and 94 (±15) for fractionations (Gy) of 2, 2.5 and
3, respectively. The foci with GS 7: 4+3 needed
significantly higher doses than the foci with GS 7: 3+4 to
destroy the tumor cells from 50% to 99.9%, at all
fractionations (Mann-Whitney test).
Conclusion
Our histopathological specimen based simulations allowed
to estimate the total doses necessary to kill the tumor
cells, depending on the fractionation. GS: 4+3 tissue
appears more radioresistant than GS:3+4 tissue.
EP-1599 Mathematical modeling of the synergistic
combination of cancer immunotherapy and
radiotherapy
C. Ceberg
1
, J. Ahlstedt
2
, H. Redebrant Nittby
3
1
Ceberg Crister, Medical Radiation Physic- Lund
University, Lund, Sweden
2
Lund University, The Rausing Laboratory, Lund, Sweden
3
Skåne University Hospital, Department of Neurosurgery,
Lund, Sweden
Purpose or Objective
Cancer immunotherapy is a promising treatment modality
that is currently under strong development with a large
number of ongoing pre-clinical and clinical studies. In an
attempt to improve the treatment efficacy combinatorial
strategies are explored, and the combination of
immunotherapy and radiotherapy is of particular interest,
since more than half of all cancer patients already receive
radiotherapy as part of their treatment. It is well known
that radiation has immunomodulatory effects. In addition
to killing off tumor cells as well as immune effector cells,
radiation also affects the release of tumor antigens, the
dendritic cell activity and antigen presentation, the