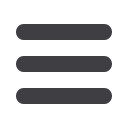

149
SUMMARY
Cochlear implants, which provide hearing for the deaf, have evolved in recent decades from single-channel
implants to multichannel implants that are able to restore speech perception abilities for many. Cochlear
implantation has eased communication with the hearing world and has greatly facilitated language
development in children. However, considerable variation in performance exists among subjects, and speech
perception in background noise continues to be troublesome for most, if not all cochlear implant recipients.
Cochlear implants consist of external and internal parts. The external part contains a microphone to pick
up the sound signal. The sound signal is then processed in a speech processor. Basically, the speech processor
codes the auditory signal based on separate frequency bands. Subsequently, the coded signal is sent through
the skin to the receiver of the internal part by a transmitter coil. The received signal is then passed to the
electrode array, which is located in the scala tympani of the cochlea. The signal leaving the different electrode
contacts stimulates the auditory nerve fibers present in that portion of the cochlea. Cochlear implants form
an interface between an audio signal and the nerve fibers of the deaf ear. This thesis focuses on optimizing
the way in which the incoming speech signal is transferred to the excitable neural elements in the cochlea.
Chapter 1
provides a general introduction to the matters discussed in this thesis. It gives a historical
overview of the developmental steps of cochlear implants, and it presents the outline of the present thesis.
Chapter 2
describes a study that analyzes the potential benefit of preprocessing the incoming signal to
increase the signal to noise ratio for cochlear implant recipients. For thirteen cochlear implant patients,
speech perception using directional microphones was compared with speech perception using an
omnidirectional microphone. To mimic real-life situations, speech in noise was presented in a specially
designed environment with a diffuse noise field. With assistive directional microphones, speech recognition
in background noise improved substantially, and speech recognition in quiet was not affected. At an SNR of
0 dB, the average CVC scores improved from 45% for the headpiece microphone to 67% and 62% for the
TX3 Handymic and the Linkit directional microphones, respectively. The speech reception threshold (SRT)
improved by 8.2 dB with the TX3 Handymic and 5.9 dB with the Linkit, compared with the headpiece. It
is concluded that these assistive microphones will allow users to understand speech in noisy environments
with greater ease.
Chapter 3
studies several clinical aspects of the use of perimodiolar electrodes. It compares the data
of 25
patients, who were implanted with a Clarion HiFocus 1 with a silastic positioner, with that of 20 patients
in whom the same implant was used, but without positioner.
After one year of implant use, the patients
who were implanted with a positioner showed a significantly better speech perception (67 vs 45% words
correct on CVC words in quiet, p < 0.01), while the pre-operative characteristics were comparable between
the groups. CT scans showed that the positioner brought the electrode closer to the modiolus basally,
whereas apically, no difference in distances from the modiolus was present. Additionally, the positioner