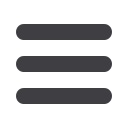

A GLOBAL OUTLOOK ON METHANE GAS HYDRATES
71
concern is that the free gas cooled by the endothermy of gas
hydrate dissociation and by effects associated with the pres-
sure reduction and the high gas velocities in the vicinity of the
well (the Joule-Thompson effect) can potentially lead to the ref-
ormation of gas hydrate in the well bore or production tubing,
causing serious operational problems. Examples of unwanted
hydrate formation plugging pipelines or processing streams
are well known in the oil and gas industry and have caused
costly shutdowns, sometimes for months. Technologies rou-
tinely employed to reduce this problem are referred to as flow
assurance. They include injection of low dose gas hydrate in-
hibitors, adding heat to the system, or generating a gas hydrate
slurry that can be flushed out.
3.4.2
Heating the reservoir
The objective of the reservoir-heating technique is to increase
the temperature within the reservoir beyond the localized
pressure-temperature threshold for gas hydrate stability. The
only full-scale field production test using this technique was
conducted at the Mallik site as part of the 2002 gas hydrate
To prove applicability of the depressurization technique as a
feasible productionmethod inmethane hydrates in deepwater
sediments, Japan Oil, Gas and Metals National Corporation
(JOGMEC) conducted the first offshore production test off
the coasts of Honshu island. A drilling vessel “Chikyu” was
employed for the field program that was started in early 2012
with drilling of production and monitoring boreholes and
intensive data acquisitions, and the flow test (Yamamoto
et
al.
, 2014). OnMarch 12, 2013, JOGMEC confirmed production
of methane gas estimated from methane hydrate layers after
lowering the bottomhole pressure of the production hole. The
pressure was reduced from the original pressure of 13.5MPa to
4.5MPa, and approximately 120,000Sm
3
of methane gas was
produced until sand production forced to terminate the flow
on March 18. Data from this program is still being analyzed
by JOGMEC, in partnership with the National Institute of
Advanced Industrial Science and Technology (AIST).
Box 3.3
Testing production in offshore
Japan setting: The Nankai Trough
production-testing program (Dallimore and Collett 2005).
The test lasted approximately five days. Hot brine (70°C at
surface / 50°C at formation depth) was circulated across a
13-metre perforated test interval. Bottomhole flowing pres-
sure was maintained slightly above formation pressure.
Thus the test permitted assessment of the efficiency of heat
conduction into the formation (that is, with no direct heat
transfer by formation fluids). With only 500 cubic metres of
gas produced over the entire testing period, the 2002 Mallik
test was not particularly productive. However, the objective
of the test was to demonstrate the feasibility of producing
gas that originated indisputably from hydrate deposits, rath-
er than the maximization of such production. It suggested
that thermal heating alone is likely to be a comparatively in-
efficient and expensive way to produce gas hydrates over the
long term. Moridis and Reagan (2007a) and Moridis
et al.
(2009) demonstrated through numerical simulation studies
that thermal stimulation is thousands of times less effective
than depressurization as a dissociation-inducing method for
gas production from hydrates.
Research continues into developing downhole-heating tech-
niques that require lower direct-energy input and provide
more effective heating of the formation (Schicks
et al.
2011).
Downhole heating may be beneficial, in some reservoir set-
tings, to overcome endothermic cooling of the formation
caused by gas hydrate dissociation and/or to manage the
temperature regime of the gas stream to prevent re-forma-
tion of gas hydrates in the vicinity of the wellbore and inside
the tubing. For certain reservoir conditions, a combination
of reservoir depressurization and supplementary in situ
heating might be optimal for sustaining gas hydrate pro-
duction over the longer term (Moridis and Reagan 2007b;
Moridis
et al.
2009).
3.4.3
Chemical stimulation
Gas hydrate production by chemical stimulation involves
the manipulation of gas hydrate phase-equilibrium condi-
tions by injecting dissociation-inducing chemicals, such as
salts and alcohols, into the reservoir. These chemicals alter
the energy potential of water in contact with the solid gas
hydrate phase, causing dissociation. This approach has been
used for decades to maintain flow assurance in gas wells and