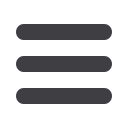

S
eptember
2009
133
›
new bend die. A third model was simulated without an applied boost
load, using the original bend die profile. The same friction and other
conditions were applied to the three models except as mentioned
above. The bend OD wall thinning ratio, ID wall thickening ratio,
and ovality predicted by the three models are plotted in terms of the
bend angle. As shown in Figure 5, the clamp die, or the head of the
tube is considered the start of the bending angle (0°). The tail of the
tube is considered the end of the bending (180°).
With no boost load, the OD wall thinning ratio is about 16%, as
shown in Figure 6. The distribution of the OD wall around the
bend was predicted to be fairly uniform. With the optimised boost
load schedule, the OD wall thinning was effectively controlled for
bending angles less than 150°. The OD wall thickness is directly
affected by the boost load. The higher boost load at the beginning
of the bending process is predicted to result in a low amount of OD
wall thinning, around 3% to 4%. However, as bending progresses
past the 90° position, the maximum boost load is limited due to the
possibility of the tube detaching from the die, and the amount of OD
wall thinning is predicted to increase. The OD wall thinning ratio
predicted from the FEA model is about 10% close to the tube tail.
The two bend die geometries are predicted to result in similar levels
of wall thinning at the OD of the bend.
Without boost load, the wall thickening at the bend ID is about 20%,
as shown in Figure 7. With boost load, the ID wall thickening is
much greater, with values of 35 to over 40%. The bend die profile
is also predicted to affect the ID wall thickness and the ovality
significantly, as shown in both Figures 7 and 8. It is interesting that
for this specific case, the bend die profile did not affect the OD wall
thickness significantly while it did affect the other two geometric
characteristics of the bend.
With the new bend die, the ID wall thickening is predicted to be
about 40%, versus 35% from the original die. The ovality ratio is
shown in Figure 8; a positive ratio means the vertical OD is greater
than the horizontal OD. With no boost load, the ovality is greater
than with a boost load and the tube slightly collapses, as signified
by the positive sign for the ovality ratio. When a boost load is used,
the ovality is reduced and the sign is reversed. Besides the effect
of the bend wall thickness, the bend die geometry has an impact on
ovality. The effect of the bend die on the ovality ratio should be a
combined effect of boost load and the pressure die profile as well.
Using the research data to program
the tube bender
The results from FEA simulations can be effectively used to set-up a
tube bending process and improve the quality of the bend geometry
from the first bend trial. However, to be successful as a design tool,
the model must be validated against experimental results to be
certain that the model variables are equivalent to the actual process
variables, namely friction, tube strength, tube dimensions and the
variations of these parameters. The following example compares
the results of the FE model presented above and tube bending
experiments.
Machine settings
A Pines CNC 150 HD tube bender, shown in Photo 2, was available
for the practical tests. The CNC 150 HD has programmable booster
pressures and 180 pressure zones.
Figure 6
:
OD wall thinning ratio distribution
Figure 7
:
ID wall thickening ratio distribution
Figure 8
:
Ovality distribution
Photo 2
:
Pines CNC 150 HD tube bender
Angle (Degree)
Angle (Degree)
ID Wall Thickening Ratio
OD Wall Thinning Ratio
Ovality Ratio
Angle (Degree)