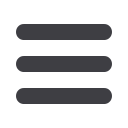

S432
ESTRO 36
_______________________________________________________________________________________________
different equipment to simulated machine errors and
explores the role of different planning approaches to this
Material and Methods
VMAT plans were generated for a selected patient in
Pinnacle
3
at three institutions, as per their local protocol.
An automated VMAT plan was also generated by institution
3 using Pinnacle
3
Autoplanning. Simulated machine errors
were deliberately introduced to the plans utilising Python.
These included collimator (°), MLC field size (mm) and
MLC shift (mm) errors of 5, 2, 1, -1, -2 and -5 units. Error-
introduced plans were then recalculated and reviewed.
The DVH metrics listed in Table 1 were deemed
unacceptable if their differences relative to the relevant
baseline plan were above the tolerances listed. Plans were
considered unacceptable if any one or more of the limits
were exceeded.
Table 1. DVH metrics and limits.
For each error type (i.e. in Collimator, C; MLC shift,
S; MLC Field Size, FS), the smallest error plans that were
deemed unacceptable were delivered within the given
institution; on an Elekta Linac, measured using an
Arccheck for institutions 1 and 3, and on a Varian Linac ,
measured using a Delta4 for institution 2. Gamma analysis
was performed in SNC Patient version 6.6 or Delta4
software respectively, utilising a 3%/3mm and 2%/2mm
global gamma pass rate (10% isodose threshold with
correction off). Before each set of measurements, MLC
checks and a complex benchmark patient test were used
to ensure the Linacs' performances were within normal
range.
Results
The global 3%/3mm gamma pass is able to detect the
majority of unacceptable plans; however some plans with
significant errors still pass. Interestingly the error type/s
that passed differed at differing institutions (Figure 1).
Figure 1. The smallest error plans (including Collimator
(C), MLC shift (S), and MLC Field Size (FS) error) which
exceeded global gamma pass rates. Errors detected if the
gamma pass rate was < 95% (for 3%/3mm) or <88%
(2%/2mm). Plans that passed are illustrated above the red
lines. Negative MLC errors were not performed at
Institution 2, due to differing equipment.
The automated VMAT plans from institution 3 were similar
in pass rate to the manually planned VMAT for collimator
errors, despite the difference (higher magnitude for
manual VMAT plans) in error magnitude. This could be
caused by the higher MLC modulation in the automated
plans.
Conclusion
Not all deliberately introduced errors were discovered for
VMAT plans using a typical 3%/3mm global gamma pass
rate (for 10% threshold with correction off). Consistency
between institutions was low for plans assessed utilising
differing devices and software. A 2%/2mm global analysis
was most sensitive to errors.
PO-0809 A 3D polymer gel dosimeter coupled to a
patient-specific anthropomorphic phantom for proton
therapy
M. Hillbrand
1
, G. Landry
2
, G. Dedes
2
, E.P. Pappas
3
, G.
Kalaitzakis
4
, C. Kurz
2
, F. Dörringer
2
, K. Kaiser
2
, M. Würl
2
,
F. Englbrecht
2
, O. Dietrich
5
, D. Makris
3
, E. Pappas
6
, K.
Parodi
2
1
Rinecker Proton Therapy Center, Medical Physics,
Munich, Germany
2
Ludwig-Maximilians-Universität München, Department
of Medical Physics, Munich, Germany
3
National and Kapodistrian University of Athens, Medical
Physics Laboratory- Medical School, Athens, Greece
4
University of Crete, Department of Medical Physics,
Heraklion, Greece
5
Ludwig-Maximilians-Universität München, Department
of Radiology, Munich, Germany
6
Technological Educational Institute, Radiology &
Radiotherapy Department, Athens, Greece
Purpose or Objective
The high conformity of proton therapy (PT) dose
distributions, attributed to protons stopping in the target,
is also the main source of uncertainty of the modality. PT
is sensitive to errors in relative stopping power to water
(RSP) uncertainties and to density changes caused by
organ motion. The ability to verify PT dose distributions in
3D with a high resolution is therefore a key component of
a safe and effective PT program. Existing 2D dosimetric
methods suffer from shortcomings attributed to LET
dependence, positioning uncertainties, limited spatial
resolution and their intrinsic 2D nature. Recent advances
in polymer gel dosimetry coupled to 3D printing
technology have enabled the production of high
resolution, patient specific dosimetry phantoms. So far
this approach has not been tested for PT.
Material and Methods
A 3D-printed hollow head phantom derived from real CT
data was filled with VIPAR6 polymer gel and CT scanned
for pencil beam scanning (PBS) treatment planning,
following RSP characterization of the gel and the 3D
printer bone mimicking material (see Figure 1). All
irradiations of phantoms were carried out at the Rinecker
Proton Therapy Center in Munich, which is dedicated for
PBS. An anterior oblique SFUD plan was used to cover a
centrally located cerebral PTV, following the standard
operating procedures of the PT facility. The field was
crossing the paranasal sinuses (see Figure 2A) to test the
TPS modelling of heterogeneities. 3D maps of the T2
relaxation time were obtained from subsequent MR
scanning of the phantom and were converted to relative
dose. The dose response linearity and proton range were
verified using separate mono-energetic irradiations of
cubic phantoms filled with gel from the same batch.
Relative dose distributions were compared to the TPS
predictions using gamma analysis.